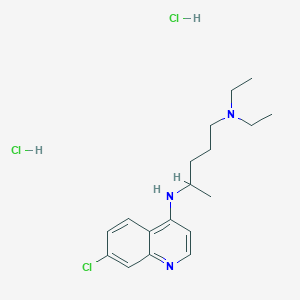
Chloroquine hydrochloride
説明
Chloroquine hydrochloride (CQ) is a 4-aminoquinoline derivative first synthesized in 1934 and widely used for malaria prophylaxis and treatment due to its ability to disrupt Plasmodium parasite digestion in red blood cells . It accumulates in lysosomes as a weak base, inhibiting heme detoxification in malaria parasites . Beyond antimalarial use, CQ has applications in autoimmune diseases (e.g., lupus, rheumatoid arthritis) and cancer therapy, where it inhibits autophagy to sensitize tumor cells to chemotherapy .
特性
IUPAC Name |
4-N-(7-chloroquinolin-4-yl)-1-N,1-N-diethylpentane-1,4-diamine;dihydrochloride | |
---|---|---|
Source | PubChem | |
URL | https://pubchem.ncbi.nlm.nih.gov | |
Description | Data deposited in or computed by PubChem | |
InChI |
InChI=1S/C18H26ClN3.2ClH/c1-4-22(5-2)12-6-7-14(3)21-17-10-11-20-18-13-15(19)8-9-16(17)18;;/h8-11,13-14H,4-7,12H2,1-3H3,(H,20,21);2*1H | |
Source | PubChem | |
URL | https://pubchem.ncbi.nlm.nih.gov | |
Description | Data deposited in or computed by PubChem | |
InChI Key |
PCFGECQRSMVKCC-UHFFFAOYSA-N | |
Source | PubChem | |
URL | https://pubchem.ncbi.nlm.nih.gov | |
Description | Data deposited in or computed by PubChem | |
Canonical SMILES |
CCN(CC)CCCC(C)NC1=C2C=CC(=CC2=NC=C1)Cl.Cl.Cl | |
Source | PubChem | |
URL | https://pubchem.ncbi.nlm.nih.gov | |
Description | Data deposited in or computed by PubChem | |
Molecular Formula |
C18H28Cl3N3 | |
Source | PubChem | |
URL | https://pubchem.ncbi.nlm.nih.gov | |
Description | Data deposited in or computed by PubChem | |
DSSTOX Substance ID |
DTXSID501026664 | |
Record name | Chloroquine hydrochloride | |
Source | EPA DSSTox | |
URL | https://comptox.epa.gov/dashboard/DTXSID501026664 | |
Description | DSSTox provides a high quality public chemistry resource for supporting improved predictive toxicology. | |
Molecular Weight |
392.8 g/mol | |
Source | PubChem | |
URL | https://pubchem.ncbi.nlm.nih.gov | |
Description | Data deposited in or computed by PubChem | |
CAS No. |
3545-67-3 | |
Record name | Chloroquine hydrochloride [USP] | |
Source | ChemIDplus | |
URL | https://pubchem.ncbi.nlm.nih.gov/substance/?source=chemidplus&sourceid=0003545673 | |
Description | ChemIDplus is a free, web search system that provides access to the structure and nomenclature authority files used for the identification of chemical substances cited in National Library of Medicine (NLM) databases, including the TOXNET system. | |
Record name | Chloroquine hydrochloride | |
Source | EPA DSSTox | |
URL | https://comptox.epa.gov/dashboard/DTXSID501026664 | |
Description | DSSTox provides a high quality public chemistry resource for supporting improved predictive toxicology. | |
Record name | N4-(7-chloro-4-quinolyl)-N1,N1-diethylpentane-1,4-diamine dihydrochloride | |
Source | European Chemicals Agency (ECHA) | |
URL | https://echa.europa.eu/substance-information/-/substanceinfo/100.020.539 | |
Description | The European Chemicals Agency (ECHA) is an agency of the European Union which is the driving force among regulatory authorities in implementing the EU's groundbreaking chemicals legislation for the benefit of human health and the environment as well as for innovation and competitiveness. | |
Explanation | Use of the information, documents and data from the ECHA website is subject to the terms and conditions of this Legal Notice, and subject to other binding limitations provided for under applicable law, the information, documents and data made available on the ECHA website may be reproduced, distributed and/or used, totally or in part, for non-commercial purposes provided that ECHA is acknowledged as the source: "Source: European Chemicals Agency, http://echa.europa.eu/". Such acknowledgement must be included in each copy of the material. ECHA permits and encourages organisations and individuals to create links to the ECHA website under the following cumulative conditions: Links can only be made to webpages that provide a link to the Legal Notice page. | |
Record name | CHLOROQUINE HYDROCHLORIDE | |
Source | FDA Global Substance Registration System (GSRS) | |
URL | https://gsrs.ncats.nih.gov/ginas/app/beta/substances/NT0J0815S5 | |
Description | The FDA Global Substance Registration System (GSRS) enables the efficient and accurate exchange of information on what substances are in regulated products. Instead of relying on names, which vary across regulatory domains, countries, and regions, the GSRS knowledge base makes it possible for substances to be defined by standardized, scientific descriptions. | |
Explanation | Unless otherwise noted, the contents of the FDA website (www.fda.gov), both text and graphics, are not copyrighted. They are in the public domain and may be republished, reprinted and otherwise used freely by anyone without the need to obtain permission from FDA. Credit to the U.S. Food and Drug Administration as the source is appreciated but not required. | |
準備方法
合成経路と反応条件
クロロキン塩酸塩は、複数段階のプロセスによって合成されます。 合成には通常、4,7-ジクロロキノリンとジエチルアミンを反応させてN4-(7-クロロ-4-キノリル)-N1,N1-ジエチル-1,4-ペンタンジアミンを生成する反応が含まれます 。 この中間体はその後、塩酸と反応させてクロロキン塩酸塩を生成します。
工業的生産方法
クロロキン塩酸塩の工業生産は、同様の合成経路を大規模に行います。 このプロセスには、水層の分離、酢酸イソプロピルの蒸留、低温での結晶化が含まれ、最終生成物が得られます 。 その後、生成物を乾燥させて、医薬品に使用できる白色の乾燥した生成物を得ます .
化学反応の分析
科学研究における用途
クロロキン塩酸塩は、科学研究において幅広い用途があります。
科学的研究の応用
Autoimmune Diseases
Chloroquine hydrochloride is widely used in the management of autoimmune diseases such as systemic lupus erythematosus and rheumatoid arthritis. Its immunomodulatory effects help reduce inflammation and prevent disease flares.
Case Study: Systemic Lupus Erythematosus
A study indicated that chloroquine is effective in reducing disease activity in patients with systemic lupus erythematosus, especially in those who are pregnant. The drug's ability to modulate immune responses contributes to improved clinical outcomes.
Viral Infections
Chloroquine has gained attention for its potential efficacy against viral infections, notably during the COVID-19 pandemic.
Research Findings on COVID-19
Clinical trials have explored chloroquine's role in treating COVID-19, with some studies suggesting it may inhibit viral replication and modulate the immune response to prevent cytokine storms. However, results have been mixed, necessitating further research to establish definitive efficacy.
Study | Findings | Sample Size | Outcome |
---|---|---|---|
Study A | Significant reduction in viral load after treatment | 120 patients | 90% negative tests after treatment |
Study B | No significant difference compared to control group | 11 patients | Higher mortality rate observed |
Cancer Treatment
Chloroquine's potential as an adjunct therapy in oncology has been investigated due to its ability to enhance the effects of chemotherapy.
作用機序
類似化合物との比較
Structural and Pharmacological Differences
Hydroxychloroquine (HCQ)
HCQ differs from CQ by a single hydroxyl group (-OH) on the side chain, reducing toxicity while retaining antimalarial and immunomodulatory efficacy . Structural variations alter binding targets: HCQ interacts with CYS 145 and SER 144 in proteins, whereas CQ binds MET 165 and GLN 189 . This difference may explain HCQ’s preferential use in autoimmune conditions due to better tolerability .
Amodiaquine Hydrochloride
Amodiaquine shares a similar quinoline backbone but includes a Mannich base side chain. This structural modification enhances activity against some chloroquine-resistant Plasmodium strains but increases hepatotoxicity and agranulocytosis risks .
Table 1: Structural and Pharmacological Comparison
Efficacy Against Resistant Strains
CQ resistance in Plasmodium falciparum is linked to mutations in the PfCRT gene.
Synergistic Combinations :
- Fluoxetine Hydrochloride : At 500 nM, fluoxetine reduced CQ resistance by 66% in vitro (vs. 61% with verapamil) by modulating drug efflux .
- Verapamil Hydrochloride : A chemosensitizer that restores CQ activity in resistant strains by inhibiting PfCRT-mediated efflux .
Table 2: Combination Therapies and Synergistic Effects
生物活性
Pharmacokinetics
Chloroquine is well-absorbed after oral administration, with bioavailability ranging from 52% to 114% depending on formulation. Key pharmacokinetic parameters include:
Antimalarial Activity
Chloroquine remains effective against sensitive strains of Plasmodium falciparum, although resistance has emerged in some regions. Its efficacy is attributed to its ability to disrupt the parasite's digestive vacuole.
Antiviral Activity
Recent studies have highlighted chloroquine's potential against various viral infections:
- Zika Virus : Research demonstrated that chloroquine reduces Zika virus replication in vitro without cytotoxic effects, suggesting its role as a therapeutic agent against this virus.
- COVID-19 : During the COVID-19 pandemic, chloroquine was investigated for its antiviral properties against SARS-CoV-2. While initial studies showed promise, subsequent clinical trials have yielded mixed results regarding its efficacy.
Anticancer Properties
Chloroquine's ability to inhibit autophagy has led to its exploration as an adjunctive treatment in cancer therapy. It enhances the efficacy of chemotherapeutic agents by preventing cancer cells from utilizing autophagy as a survival mechanism.
Case Studies
- Malaria Treatment : A study involving patients with uncomplicated malaria showed that chloroquine effectively reduced parasitemia within 48 hours of treatment, with a significant clinical cure rate observed.
- Zika Virus Infection : In a controlled laboratory setting, chloroquine treatment resulted in a significant decrease in Zika virus-infected cells across multiple cell types, demonstrating its potential as a preventive measure against congenital Zika syndrome.
- COVID-19 Trials : Clinical trials assessing chloroquine for COVID-19 treatment showed varied outcomes; while some indicated reduced viral load, others reported no significant benefit compared to standard care.
Adverse Effects and Considerations
Despite its therapeutic benefits, chloroquine is associated with several adverse effects:
Q & A
Basic Research Questions
Q. What analytical methods are recommended for assessing the purity and stability of chloroquine hydrochloride in pharmaceutical formulations?
- High-Performance Liquid Chromatography (HPLC) is the gold standard for purity analysis. A validated HPLC protocol should use a C18 column with a mobile phase of phosphate buffer (pH 3.0) and acetonitrile (75:25 v/v) at a flow rate of 1.0 mL/min. Detection is typically performed at 254 nm, ensuring resolution between chloroquine and its impurities (e.g., desethylchloroquine). System suitability criteria require a resolution ≥2.0 between peaks and ≤2.0% RSD for reproducibility .
Q. How is this compound’s antimalarial mechanism evaluated in in vitro models?
- Use synchronized cultures of Plasmodium falciparum in 96-well microplates with RPMI-1640 medium supplemented with human serum. Chloroquine’s IC50 is determined via dose-response curves (3.75–240 µg/mL) after 48-hour incubation. Parasitemia rates are quantified by microscopy (counting schizonts with ≥3 nuclei per 200 parasites). Include verapamil (125 µg/L) as a modulator to assess resistance reversal .
Q. What spectroscopic techniques are used to characterize this compound’s molecular interactions?
- Fourier-Transform Infrared Spectroscopy (FTIR) identifies functional groups (e.g., quinoline ring vibrations at 1590–1610 cm⁻¹). X-ray Photoelectron Spectroscopy (XPS) analyzes elemental composition and oxidation states (e.g., N 1s peaks at 399.5 eV for amine groups). These methods are critical for studying drug-metal complexes or degradation products .
Q. How is this compound’s pharmacokinetic profile assessed in preclinical models?
- Administer chloroquine intravenously (5 mg/kg) or orally (10 mg/kg) in rodent models. Collect plasma samples at intervals (0.5–24 hours) and quantify drug levels using LC-MS/MS with a lower limit of detection (LLOD) of 1 ng/mL. Key parameters include Cmax, Tmax, and half-life (t½ ~20–60 days in humans) .
Advanced Research Questions
Q. How can researchers resolve contradictory data between in vitro antimalarial efficacy and in vivo preclinical outcomes?
- Cross-validate experimental conditions: Ensure in vitro parasite strains match the genetic background of in vivo models (e.g., chloroquine-resistant P. berghei). Adjust dosing regimens to account for species-specific metabolism. Use pharmacokinetic-pharmacodynamic (PK/PD) modeling to correlate tissue drug concentrations with parasitemia suppression .
Q. What strategies optimize this compound’s bioavailability in experimental formulations?
- Develop lipid-based nanoparticles (e.g., solid lipid nanoparticles, SLNs) using hot homogenization. Characterize particle size (100–200 nm via DLS), entrapment efficiency (>85% via ultrafiltration), and in vitro release (pH 6.8 phosphate buffer, 72-hour sustained release). Compare bioavailability in rodent models using AUC0–24 ratios .
Q. How should researchers design a systematic review protocol to evaluate chloroquine’s repurposing potential (e.g., for viral infections)?
- Follow PRISMA-IPD guidelines: Define inclusion criteria (RCTs with participant-level data), extract variables (dose, demographics, outcomes), and harmonize datasets using standardized coding. Conduct cross-citation searches in Google Scholar and contact authors for unreported data. Use mixed-effects models to account for heterogeneity .
Q. What methodologies address discrepancies in chloroquine’s autophagy inhibition mechanisms across cell lines?
- Standardize assays: Use GFP-LC3 transfection to quantify autophagosome formation via fluorescence microscopy. Validate with Western blotting for LC3-II/LC3-I ratios and p62 degradation. Control for lysosomal pH (e.g., bafilomycin A1 pretreatment) and cell-specific uptake kinetics .
Q. How can researchers mitigate batch-to-batch variability in this compound synthesis?
- Implement Quality by Design (QbD) principles: Optimize reaction parameters (temperature: 60–80°C, pH: 4.5–5.5) via factorial design. Use Process Analytical Technology (PAT) tools like inline NIR spectroscopy to monitor intermediate purity. Validate synthetic routes using USP reference standards for identity testing .
Q. What statistical approaches reconcile conflicting clinical trial data on chloroquine’s efficacy (e.g., in COVID-19)?
- Perform individual participant data (IPD) meta-analysis. Adjust for covariates (age, comorbidities) using multivariate regression. Apply trial sequential analysis (TSA) to assess false-positive risks. Stratify results by dosing regimens (e.g., 500 mg BID vs. 250 mg QD) and endpoint definitions (e.g., viral clearance vs. mortality) .
Retrosynthesis Analysis
AI-Powered Synthesis Planning: Our tool employs the Template_relevance Pistachio, Template_relevance Bkms_metabolic, Template_relevance Pistachio_ringbreaker, Template_relevance Reaxys, Template_relevance Reaxys_biocatalysis model, leveraging a vast database of chemical reactions to predict feasible synthetic routes.
One-Step Synthesis Focus: Specifically designed for one-step synthesis, it provides concise and direct routes for your target compounds, streamlining the synthesis process.
Accurate Predictions: Utilizing the extensive PISTACHIO, BKMS_METABOLIC, PISTACHIO_RINGBREAKER, REAXYS, REAXYS_BIOCATALYSIS database, our tool offers high-accuracy predictions, reflecting the latest in chemical research and data.
Strategy Settings
Precursor scoring | Relevance Heuristic |
---|---|
Min. plausibility | 0.01 |
Model | Template_relevance |
Template Set | Pistachio/Bkms_metabolic/Pistachio_ringbreaker/Reaxys/Reaxys_biocatalysis |
Top-N result to add to graph | 6 |
Feasible Synthetic Routes
Featured Recommendations
Most viewed | ||
---|---|---|
Most popular with customers |
試験管内研究製品の免責事項と情報
BenchChemで提示されるすべての記事および製品情報は、情報提供を目的としています。BenchChemで購入可能な製品は、生体外研究のために特別に設計されています。生体外研究は、ラテン語の "in glass" に由来し、生物体の外で行われる実験を指します。これらの製品は医薬品または薬として分類されておらず、FDAから任何の医療状態、病気、または疾患の予防、治療、または治癒のために承認されていません。これらの製品を人間または動物に体内に導入する形態は、法律により厳格に禁止されています。これらのガイドラインに従うことは、研究と実験において法的および倫理的な基準の遵守を確実にするために重要です。