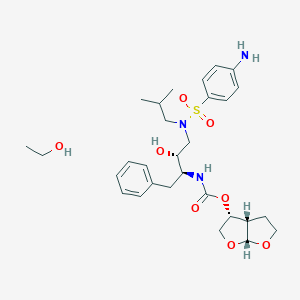
ダルナビールエタノール
概要
説明
ダラナビールエタノレートは、ヒト免疫不全ウイルス(HIV)感染症の治療に使用される第2世代プロテアーゼ阻害剤です。 プレジスタという商品名で販売されており、多くの場合、他の抗レトロウイルス剤と組み合わせて使用することで、その有効性を高めます . ダラナビールエタノレートは、標準的なHIV療法に対する抵抗に対抗する能力で知られており、HIV-1感染症の管理において重要な要素となっています .
科学的研究の応用
Darunavir ethanolate has a wide range of scientific research applications, particularly in the fields of chemistry, biology, medicine, and industry. In medicine, it is used as a key component in antiretroviral therapy for the treatment of HIV-1 infection . It is also being studied as a potential treatment for SARS-CoV-2, the virus responsible for COVID-19, due to its ability to combat the infection in vitro .
In chemistry, darunavir ethanolate is used as a model compound for studying the synthesis and optimization of protease inhibitors. Its unique structure and properties make it an ideal candidate for research in drug development and formulation .
作用機序
Safety and Hazards
生化学分析
Biochemical Properties
Darunavir Ethanolate interacts with the HIV-1 protease, an enzyme crucial for the life-cycle of the virus . It inhibits and is primarily metabolized by cytochrome P450 3A (CYP3A) isoenzymes . This interaction with CYP3A isoenzymes results in increased plasma concentrations of Darunavir Ethanolate, allowing for a lower daily dose .
Cellular Effects
Darunavir Ethanolate has a significant impact on various types of cells, particularly those infected with HIV-1. It decreases the viral load and increases CD4 cell counts, reducing the morbidity and mortality of HIV infection . It also influences cell function by inhibiting the HIV-1 protease, which prevents HIV viral replication .
Molecular Mechanism
The molecular mechanism of Darunavir Ethanolate involves blocking the action of HIV protease. By inhibiting this enzyme, Darunavir Ethanolate prevents the virus from maturing and producing infectious viral particles . This inhibition is achieved through binding interactions with the enzyme, leading to changes in gene expression and enzyme activity .
Temporal Effects in Laboratory Settings
In laboratory settings, the effects of Darunavir Ethanolate have been observed to change over time. The terminal elimination half-life (t1/2) of Darunavir Ethanolate is approximately 15 hours in the presence of ritonavir . This suggests that the drug has a relatively long duration of action, allowing for less frequent dosing .
Dosage Effects in Animal Models
The standard dosage has been carefully calibrated to maximize therapeutic benefits while minimizing potential adverse effects .
Metabolic Pathways
Darunavir Ethanolate is heavily metabolized by hepatic cytochrome enzymes, mainly CYP3A . It undergoes extensive metabolism, primarily via carbamate hydrolysis, isobutyl aliphatic hydroxylation, and aniline aromatic hydroxylation .
Transport and Distribution
Darunavir Ethanolate is highly protein-bound, indicating that it may be transported within the body bound to proteins such as albumin . This could influence its distribution within cells and tissues.
Subcellular Localization
Given its role as a protease inhibitor, it is likely to interact with the HIV-1 protease in the cytoplasm of the cell where viral replication occurs .
準備方法
合成経路と反応条件: ダラナビールエタノレートの合成は、重要な中間体の形成と最終的なカップリング反応を含む、複数のステップからなります。 一般的な方法の1つは、溶媒湿潤、熱溶融押出、スプレー乾燥技術を使用し、ダラナビールの溶解性とバイオアベイラビリティを向上させることです . このプロセスには、水溶性ポリマーでアモルファス固体分散体を調製し、賦形剤と混合して錠剤に圧縮することが含まれます .
工業的製造方法: ダラナビールエタノレートの工業的製造には、5回の分離と乾燥工程を含む、堅牢で安全なプロセスが採用されています。 このプロセスにより、高い化学収率と純度が確保され、可能なすべてのプロセス不純物は、所望の限度以下に抑えられます . この製造方法は効率的でスケーラブルであり、ダラナビールエタノレートを複数キログラム量合成することができます .
化学反応の分析
反応の種類: ダラナビールエタノレートは、酸化、還元、置換など、さまざまな化学反応を起こします。 この化合物は、主にCYP3Aである肝細胞チトクローム酵素によって、強く酸化されて代謝されます . また、カルバメート加水分解、イソブチル脂肪族ヒドロキシル化、アニリン芳香族ヒドロキシル化も起こります .
一般的な試薬と条件: ダラナビールエタノレートの反応で使用される一般的な試薬には、酸化剤、還元剤、さまざまな触媒が含まれます。反応は、通常、制御された条件下で行われ、目的の生成物が生成されるようにします。
生成される主な生成物: ダラナビールエタノレートの反応から生成される主な生成物には、さまざまなヒドロキシル化代謝物とグルクロン酸抱合代謝物が含まれます . これらの代謝物は、主に糞便と尿によって排泄されます .
科学研究アプリケーション
ダラナビールエタノレートは、特に化学、生物学、医学、産業の分野において、幅広い科学研究アプリケーションを有しています。 医学においては、HIV-1感染症の治療のための抗レトロウイルス療法の重要な成分として使用されています . また、COVID-19の原因となるウイルスであるSARS-CoV-2の潜在的な治療法として研究されており、in vitroで感染に対抗する能力があります .
化学において、ダラナビールエタノレートは、プロテアーゼ阻害剤の合成と最適化を研究するためのモデル化合物として使用されます。 そのユニークな構造と特性により、創薬と製剤研究の理想的な候補となっています .
類似化合物との比較
ダラナビールエタノレートは、アンプレナビル、インジナビル、サキナビル、ネルフィナビル、リトナビルなどの他のプロテアーゼ阻害剤と構造的に類似しています . ダラナビールエタノレートは、分子全体の残りの部分に結合する結合における立体化学を逆転させる、ユニークなビス-THF部分を持っています . この構造的特徴により、標準的なHIV療法に対する抵抗に対抗する能力が向上し、多剤耐性株のHIV-1に対してより効果的になります .
類似化合物のリスト:- アンプレナビル
- インジナビル
- サキナビル
- ネルフィナビル
- リトナビル
結論
ダラナビールエタノレートは、標準的なHIV療法に対する抵抗に対抗する能力で知られる、HIV-1感染症の治療における重要な化合物です。そのユニークな構造と特性により、化学、生物学、医学、産業など、さまざまな分野における科学研究の重要な対象となっています。この化合物の堅牢な合成方法と幅広い用途は、HIV / AIDSとの闘いにおいてその重要性を強調しています。
特性
IUPAC Name |
[(3aS,4R,6aR)-2,3,3a,4,5,6a-hexahydrofuro[2,3-b]furan-4-yl] N-[(2S,3R)-4-[(4-aminophenyl)sulfonyl-(2-methylpropyl)amino]-3-hydroxy-1-phenylbutan-2-yl]carbamate;ethanol | |
---|---|---|
Source | PubChem | |
URL | https://pubchem.ncbi.nlm.nih.gov | |
Description | Data deposited in or computed by PubChem | |
InChI |
InChI=1S/C27H37N3O7S.C2H6O/c1-18(2)15-30(38(33,34)21-10-8-20(28)9-11-21)16-24(31)23(14-19-6-4-3-5-7-19)29-27(32)37-25-17-36-26-22(25)12-13-35-26;1-2-3/h3-11,18,22-26,31H,12-17,28H2,1-2H3,(H,29,32);3H,2H2,1H3/t22-,23-,24+,25-,26+;/m0./s1 | |
Source | PubChem | |
URL | https://pubchem.ncbi.nlm.nih.gov | |
Description | Data deposited in or computed by PubChem | |
InChI Key |
QWSHKNICRJHQCY-VBTXLZOXSA-N | |
Source | PubChem | |
URL | https://pubchem.ncbi.nlm.nih.gov | |
Description | Data deposited in or computed by PubChem | |
Canonical SMILES |
CCO.CC(C)CN(CC(C(CC1=CC=CC=C1)NC(=O)OC2COC3C2CCO3)O)S(=O)(=O)C4=CC=C(C=C4)N | |
Source | PubChem | |
URL | https://pubchem.ncbi.nlm.nih.gov | |
Description | Data deposited in or computed by PubChem | |
Isomeric SMILES |
CCO.CC(C)CN(C[C@H]([C@H](CC1=CC=CC=C1)NC(=O)O[C@H]2CO[C@@H]3[C@H]2CCO3)O)S(=O)(=O)C4=CC=C(C=C4)N | |
Source | PubChem | |
URL | https://pubchem.ncbi.nlm.nih.gov | |
Description | Data deposited in or computed by PubChem | |
Molecular Formula |
C29H43N3O8S | |
Source | PubChem | |
URL | https://pubchem.ncbi.nlm.nih.gov | |
Description | Data deposited in or computed by PubChem | |
DSSTOX Substance ID |
DTXSID70979794 | |
Record name | Hexahydrofuro[2,3-b]furan-3-yl hydrogen {4-[(4-aminobenzene-1-sulfonyl)(2-methylpropyl)amino]-3-hydroxy-1-phenylbutan-2-yl}carbonimidate--ethanol (1/1) | |
Source | EPA DSSTox | |
URL | https://comptox.epa.gov/dashboard/DTXSID70979794 | |
Description | DSSTox provides a high quality public chemistry resource for supporting improved predictive toxicology. | |
Molecular Weight |
593.7 g/mol | |
Source | PubChem | |
URL | https://pubchem.ncbi.nlm.nih.gov | |
Description | Data deposited in or computed by PubChem | |
CAS No. |
635728-49-3, 635728-39-1 | |
Record name | Darunavir ethanolate | |
Source | ChemIDplus | |
URL | https://pubchem.ncbi.nlm.nih.gov/substance/?source=chemidplus&sourceid=0635728493 | |
Description | ChemIDplus is a free, web search system that provides access to the structure and nomenclature authority files used for the identification of chemical substances cited in National Library of Medicine (NLM) databases, including the TOXNET system. | |
Record name | Hexahydrofuro[2,3-b]furan-3-yl hydrogen {4-[(4-aminobenzene-1-sulfonyl)(2-methylpropyl)amino]-3-hydroxy-1-phenylbutan-2-yl}carbonimidate--ethanol (1/1) | |
Source | EPA DSSTox | |
URL | https://comptox.epa.gov/dashboard/DTXSID70979794 | |
Description | DSSTox provides a high quality public chemistry resource for supporting improved predictive toxicology. | |
Record name | DARUNAVIR ETHANOLATE | |
Source | European Chemicals Agency (ECHA) | |
URL | https://echa.europa.eu/information-on-chemicals | |
Description | The European Chemicals Agency (ECHA) is an agency of the European Union which is the driving force among regulatory authorities in implementing the EU's groundbreaking chemicals legislation for the benefit of human health and the environment as well as for innovation and competitiveness. | |
Explanation | Use of the information, documents and data from the ECHA website is subject to the terms and conditions of this Legal Notice, and subject to other binding limitations provided for under applicable law, the information, documents and data made available on the ECHA website may be reproduced, distributed and/or used, totally or in part, for non-commercial purposes provided that ECHA is acknowledged as the source: "Source: European Chemicals Agency, http://echa.europa.eu/". Such acknowledgement must be included in each copy of the material. ECHA permits and encourages organisations and individuals to create links to the ECHA website under the following cumulative conditions: Links can only be made to webpages that provide a link to the Legal Notice page. | |
Record name | DARUNAVIR ETHANOLATE | |
Source | FDA Global Substance Registration System (GSRS) | |
URL | https://gsrs.ncats.nih.gov/ginas/app/beta/substances/33O78XF0BW | |
Description | The FDA Global Substance Registration System (GSRS) enables the efficient and accurate exchange of information on what substances are in regulated products. Instead of relying on names, which vary across regulatory domains, countries, and regions, the GSRS knowledge base makes it possible for substances to be defined by standardized, scientific descriptions. | |
Explanation | Unless otherwise noted, the contents of the FDA website (www.fda.gov), both text and graphics, are not copyrighted. They are in the public domain and may be republished, reprinted and otherwise used freely by anyone without the need to obtain permission from FDA. Credit to the U.S. Food and Drug Administration as the source is appreciated but not required. | |
Retrosynthesis Analysis
AI-Powered Synthesis Planning: Our tool employs the Template_relevance Pistachio, Template_relevance Bkms_metabolic, Template_relevance Pistachio_ringbreaker, Template_relevance Reaxys, Template_relevance Reaxys_biocatalysis model, leveraging a vast database of chemical reactions to predict feasible synthetic routes.
One-Step Synthesis Focus: Specifically designed for one-step synthesis, it provides concise and direct routes for your target compounds, streamlining the synthesis process.
Accurate Predictions: Utilizing the extensive PISTACHIO, BKMS_METABOLIC, PISTACHIO_RINGBREAKER, REAXYS, REAXYS_BIOCATALYSIS database, our tool offers high-accuracy predictions, reflecting the latest in chemical research and data.
Strategy Settings
Precursor scoring | Relevance Heuristic |
---|---|
Min. plausibility | 0.01 |
Model | Template_relevance |
Template Set | Pistachio/Bkms_metabolic/Pistachio_ringbreaker/Reaxys/Reaxys_biocatalysis |
Top-N result to add to graph | 6 |
Feasible Synthetic Routes
Q1: How does Darunavir exert its antiviral activity?
A1: Darunavir functions as a protease inhibitor, specifically targeting the HIV-1 protease enzyme. [] This enzyme is crucial for the virus's life cycle, playing a vital role in the cleavage of viral polyproteins into functional proteins necessary for viral maturation. [] By binding to the active site of HIV-1 protease, Darunavir prevents the enzyme from carrying out this essential function, effectively inhibiting viral replication. [, , ]
Q2: What are some alternative treatment strategies being considered when Darunavir resistance emerges?
A4: Research indicates that combination therapies, incorporating other antiretroviral agents with different mechanisms of action, can provide a more robust response. [, , ] Clinical trials have shown promising results with Darunavir used in conjunction with newer antiretroviral drugs like Etravirine (a non-nucleoside reverse transcriptase inhibitor) and Raltegravir (an integrase inhibitor). [, ] These combinations aim to target multiple stages of the viral life cycle, suppressing viral replication more effectively and potentially mitigating the risk of resistance development.
Q3: How does Darunavir behave within the body after administration?
A5: Darunavir is primarily metabolized by the cytochrome P450 3A (CYP3A) enzymes in the liver. [] To enhance its pharmacokinetic profile, Darunavir is co-administered with low-dose Ritonavir, a potent CYP3A inhibitor. [, , ] Ritonavir "boosts" Darunavir's plasma concentrations by inhibiting its metabolism, resulting in increased drug exposure and allowing for lower, less frequent dosing. [, ]
Q4: Are there any challenges related to Darunavir's pharmacokinetics in specific populations?
A6: Studies show that Darunavir exhibits pharmacokinetic variability across different demographics. Research indicates lower Darunavir exposure in pregnant women compared with postpartum, emphasizing the need for close monitoring and potential dose adjustments in this population. [, ] Additionally, Asian children have shown adequate drug exposure with standard Darunavir dosing and Ritonavir boosting, suggesting that lower Ritonavir boosting doses (common in non-Asian children) may not be necessary in this population. []
Q5: What are some research avenues for improving Darunavir-based therapies?
A8: Future research focuses on enhancing Darunavir's efficacy and accessibility. This includes developing strategies to overcome resistance, optimizing dosing regimens for specific populations like pregnant women and children, exploring novel drug delivery systems, and investigating the potential of Darunavir in combination with other antiretroviral agents. [, , , , , ]
Q6: Are there ongoing investigations into optimizing treatment strategies using Darunavir?
A9: Yes, ongoing research explores innovative approaches for maximizing Darunavir's therapeutic benefits. One area of focus is dose reduction strategies for maintaining viral suppression in patients already stable on Darunavir therapy. [] Additionally, studies are investigating the efficacy and safety of Darunavir/Ritonavir monotherapy at lower doses in specific patient populations, aiming to minimize treatment burden while maintaining viral suppression. [] These research endeavors highlight the ongoing efforts to refine Darunavir-based treatment strategies for improved patient outcomes.
試験管内研究製品の免責事項と情報
BenchChemで提示されるすべての記事および製品情報は、情報提供を目的としています。BenchChemで購入可能な製品は、生体外研究のために特別に設計されています。生体外研究は、ラテン語の "in glass" に由来し、生物体の外で行われる実験を指します。これらの製品は医薬品または薬として分類されておらず、FDAから任何の医療状態、病気、または疾患の予防、治療、または治癒のために承認されていません。これらの製品を人間または動物に体内に導入する形態は、法律により厳格に禁止されています。これらのガイドラインに従うことは、研究と実験において法的および倫理的な基準の遵守を確実にするために重要です。