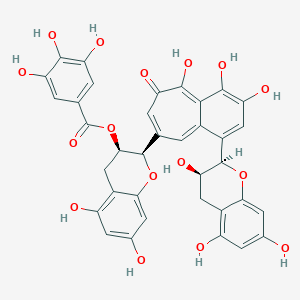
テアフラビンモノガレート
- 専門家チームからの見積もりを受け取るには、QUICK INQUIRYをクリックしてください。
- 品質商品を競争力のある価格で提供し、研究に集中できます。
説明
Theaflavins are a category of natural compounds characterized with the benzotropolone skeleton . They are found extensively in fermented tea and are regarded as the ‘golden molecules’ separated from tea . Theaflavin derivatives mainly include theaflavin (TF1), theaflavin-3-gallate (TF2A), theaflavin-3’-gallate (TF2B), and theaflavin-3,3’-digallate (TF3) .
Molecular Structure Analysis
Theaflavin monogallate is a polyphenol and a naturally available antioxidant belonging to the group of theaflavins . It has a molecular formula of C36H28O16 . Theaflavin monogallate was bound at the central cavity of lactoferrin and formed hydrogen bonds with Gln89, Tyr192, Lys301, Ser303, Gln87, and Val250 of lactoferrin .Chemical Reactions Analysis
Theaflavin monogallate shows a significant affinity towards lactoferrin with a binding constant of ∼10^4 –10^5 M^−1 at different temperatures . ANS binding shows that the binding of polyphenol resulted in the burial of hydrophobic domains of lactoferrin .Physical and Chemical Properties Analysis
Theaflavins have low bioavailability due to their high molecular weight and large polar surface area and thus require modifications before delivery . They are characterized with the structure of benzotropolone .科学的研究の応用
糖尿病管理
テアフラビンモノガレートは、血糖降下作用を有することが示されており、境界型2型糖尿病の食事療法戦略の一部として有益となる可能性があります。 これには、食後高血糖の調節が含まれます .
癌研究
研究によると、テアフラビンモノガレートは細胞増殖に異なる影響を与えることが示されており、癌治療の開発において重要となる可能性があります。 これらの化合物は、癌細胞の増殖を阻害する可能性について研究されています .
抗炎症作用
テアフラビンモノガレートの抗炎症作用は、慢性炎症および関連疾患の研究において注目されています .
心臓血管の健康
テアフラビンモノガレートは、抗アテローム性動脈硬化作用に関連付けられており、心臓血管疾患の予防と治療において重要となる可能性があります .
抗菌活性
これらの化合物は、さまざまな研究で抗ウイルスと抗菌作用を示しており、新しい抗菌剤の研究において関連性があります .
歯科の健康
テアフラビンモノガレートは、抗う蝕作用の可能性について研究されており、歯科の健康管理における新しいアプローチにつながる可能性があります .
これらのアプリケーションはそれぞれ、テアフラビンモノガレートがその潜在的な利点と用途について科学研究で探求されている独自の分野を表しています。
各アプリケーションの詳細については、提供された参考文献を参照してください。
MDPI - テアフラビン合成とその機能 AACR Journals - テアフラビンモノガレートの細胞増殖に対する異なる影響
作用機序
Target of Action
Theaflavin monogallates, a major polyphenol in black tea, have been found to interact with several targets. One of the primary targets is Cox-2 , a key enzyme for prostaglandin biosynthesis . Another significant target is lactoferrin , a heme-binding multifunctional glycoprotein known for iron transportation in the blood and contributing to innate immunity .
Mode of Action
Theaflavin monogallates interact with their targets in a unique manner. For instance, theaflavin monogallates show a significant affinity towards lactoferrin, forming a non-fluorescent complex through static quenching . They bind at the central cavity of lactoferrin and form hydrogen bonds with specific residues of lactoferrin . In the case of Cox-2, theaflavin monogallates inhibit its gene expression .
Biochemical Pathways
Theaflavin monogallates affect several biochemical pathways. They inhibit the growth of certain cancerous cells, such as SV40 transformed WI38 human cells and Caco-2 colon cancer cells, by inducing apoptosis . They also block the serum-induced Cox-2 gene expression at both mRNA and protein level .
Pharmacokinetics
It is known that theaflavin monogallates show a significant affinity towards lactoferrin, suggesting potential implications for their absorption, distribution, metabolism, and excretion (adme) properties .
Result of Action
Theaflavin monogallates exhibit differential growth-inhibitory and apoptotic effects toward cancerous cells . They inhibit the growth of certain cancerous cells but have little effect on the growth of their normal counterparts . They also completely block the serum-induced Cox-2 gene expression .
将来の方向性
The underlying formation mechanism of theaflavins has been proposed, and ascertainment of the several therapeutic efficiency of the family members of theaflavins would be the next step in the future . The effect of new formulations, including nanoformulation and encapsulation of theaflavins, will also be discussed to provide its modulated effect on its efficacy and bioavailability .
生化学分析
Biochemical Properties
Theaflavin monogallates interact with various enzymes and proteins. They have been found to interact with lactoferrin, a heme-binding multifunctional glycoprotein known for iron transportation in the blood . Theaflavin monogallate shows a significant affinity towards lactoferrin, forming a non-fluorescent complex .
Cellular Effects
Theaflavin monogallates have differential effects on cell growth, apoptosis, and gene expression in cancerous versus normal cells . They inhibit the growth of certain transformed cells and induce apoptosis in these cells but not in normal cells . They also inhibit cyclooxygenase (Cox)-2 gene expression .
Molecular Mechanism
Theaflavin monogallates exert their effects at the molecular level through binding interactions with biomolecules and changes in gene expression . They form hydrogen bonds with several residues of lactoferrin . Other residues are involved in hydrophobic interactions .
特性
{ "Design of the Synthesis Pathway": "The synthesis pathway of Theaflavin monogallates involves the reaction of Theaflavin with gallic acid in the presence of a catalyst.", "Starting Materials": [ "Theaflavin", "Gallic acid", "Catalyst" ], "Reaction": [ "Step 1: Dissolve Theaflavin and Gallic acid in a suitable solvent such as ethanol or methanol.", "Step 2: Add a suitable catalyst such as sulfuric acid or hydrochloric acid to the reaction mixture.", "Step 3: Heat the reaction mixture under reflux for several hours.", "Step 4: Allow the reaction mixture to cool and filter the solid product.", "Step 5: Wash the solid product with a suitable solvent to remove any impurities.", "Step 6: Dry the product under vacuum to obtain Theaflavin monogallates." ] } | |
CAS番号 |
30462-34-1 |
分子式 |
C36H28O16 |
分子量 |
716.6 g/mol |
IUPAC名 |
[(2R,3R)-5,7-dihydroxy-2-[3,4,6-trihydroxy-5-oxo-1-[(2R,3R)-3,5,7-trihydroxy-3,4-dihydro-2H-chromen-2-yl]benzo[7]annulen-8-yl]-3,4-dihydro-2H-chromen-3-yl] 3,4,5-trihydroxybenzoate |
InChI |
InChI=1S/C36H28O16/c37-14-5-20(39)18-10-26(45)35(51-27(18)7-14)17-9-25(44)33(48)30-16(17)1-12(2-24(43)32(30)47)34-29(11-19-21(40)6-15(38)8-28(19)50-34)52-36(49)13-3-22(41)31(46)23(42)4-13/h1-9,26,29,34-35,37-42,44-46,48H,10-11H2,(H,43,47)/t26-,29-,34-,35-/m1/s1 |
InChIキー |
KMJPKUVSXFVQGZ-WQLSNUALSA-N |
異性体SMILES |
C1[C@H]([C@H](OC2=CC(=CC(=C21)O)O)C3=CC(=C(C4=C3C=C(C=C(C4=O)O)[C@@H]5[C@@H](CC6=C(C=C(C=C6O5)O)O)OC(=O)C7=CC(=C(C(=C7)O)O)O)O)O)O |
SMILES |
C1C(C(OC2=CC(=CC(=C21)O)O)C3=CC(=C(C4=C(C(=O)C=C(C=C34)C5C(CC6=C(C=C(C=C6O5)O)O)OC(=O)C7=CC(=C(C(=C7)O)O)O)O)O)O)O |
正規SMILES |
C1C(C(OC2=CC(=CC(=C21)O)O)C3=CC(=C(C4=C3C=C(C=C(C4=O)O)C5C(CC6=C(C=C(C=C6O5)O)O)OC(=O)C7=CC(=C(C(=C7)O)O)O)O)O)O |
製品の起源 |
United States |
Q1: What are the primary biological effects of theaflavin monogallates?
A1: Research suggests that theaflavin monogallates exhibit several notable effects:
- Inhibition of α-amylase: Theaflavin monogallates have demonstrated potent inhibitory effects on α-amylase activity, surpassing the inhibitory capacity of other theaflavins like theaflavin and theaflavin digallate. [] This inhibition may contribute to their potential anti-diabetic effects.
- Differential effects on cell growth and apoptosis: Theaflavin monogallates selectively inhibit the growth of cancerous cells while showing minimal impact on their normal counterparts. [] This selectivity extends to inducing apoptosis in transformed cells but not in normal cells. []
- Suppression of Cox-2 gene expression: Theaflavin monogallates effectively inhibit cyclooxygenase-2 (Cox-2) gene expression, a key player in intestinal carcinogenesis. [] This suppression occurs at both mRNA and protein levels, suggesting a multi-pronged regulatory mechanism. []
Q2: How do theaflavin monogallates interact with cholesterol absorption?
A2: Studies reveal that theaflavin monogallates effectively reduce the micellar solubility of cholesterol, directly impacting its absorption in the intestines. [] This effect appears to be specific to cholesterol, as bile acid concentrations remain unaffected. [] This interaction suggests a potential role for theaflavin monogallates in managing cholesterol levels.
Q3: What is the role of theaflavin monogallates in the context of black tea's antioxidant properties?
A3: Theaflavin monogallates contribute significantly to the antioxidant properties of black tea. Research indicates they exhibit potent radical-scavenging abilities, effectively neutralizing DPPH radicals and superoxide radicals. [] While their lipid oxidation-inhibition activity might be less pronounced than some catechins, their overall contribution to black tea's antioxidant profile is undeniable. []
Q4: What insights do we have into the structural characteristics of theaflavin monogallates?
A4: Theaflavin monogallates, as their name suggests, are theaflavin molecules esterified with a single gallic acid moiety. Advanced spectroscopic techniques, such as 1H and 13C NMR, have been instrumental in elucidating the complete and unambiguous assignment of their molecular structure. [] This detailed structural understanding is crucial for understanding their interactions with other molecules and for further research.
Q5: Have there been studies on the interaction of theaflavin monogallates with proteins?
A5: Yes, recent studies have investigated the interaction of theaflavin monogallate with camel milk lactoferrin using a combination of biophysical techniques and computational modeling. [] The results indicate a spontaneous and stable complex formation, primarily driven by hydrophobic interactions and hydrogen bonding. [] This interaction suggests a potential influence on the bioavailability and functionality of both the polyphenol and the protein.
Q6: How stable are theaflavins, including theaflavin monogallates, during analysis?
A6: Studies using HPLC analysis demonstrated that theaflavins, including theaflavin monogallates, exhibit remarkable stability in decaffeinated aqueous tea extracts for extended periods. [] This stability is crucial for accurate quantification and analysis, particularly in quality control procedures for tea products. []
Q7: What are the challenges and considerations regarding the separation and purification of theaflavin monogallates?
A7: Isolating and purifying theaflavin monogallates from complex mixtures like black tea extracts can be challenging. Techniques like high-speed counter-current chromatography (HSCCC) have been employed to address this challenge. [] Achieving complete separation of theaflavin monogallates, particularly the different isomers, remains an area requiring further optimization and exploration of novel separation methodologies. []
Q8: Are there any computational studies focusing on the potential of theaflavin monogallates against viral targets?
A8: Yes, in silico studies employing molecular docking simulations have explored the potential inhibitory effects of theaflavin monogallates against key proteins of SARS-CoV-2, specifically PLpro and RdRp. [] These computational investigations provide preliminary evidence for potential antiviral activity and warrant further experimental validation. []
試験管内研究製品の免責事項と情報
BenchChemで提示されるすべての記事および製品情報は、情報提供を目的としています。BenchChemで購入可能な製品は、生体外研究のために特別に設計されています。生体外研究は、ラテン語の "in glass" に由来し、生物体の外で行われる実験を指します。これらの製品は医薬品または薬として分類されておらず、FDAから任何の医療状態、病気、または疾患の予防、治療、または治癒のために承認されていません。これらの製品を人間または動物に体内に導入する形態は、法律により厳格に禁止されています。これらのガイドラインに従うことは、研究と実験において法的および倫理的な基準の遵守を確実にするために重要です。