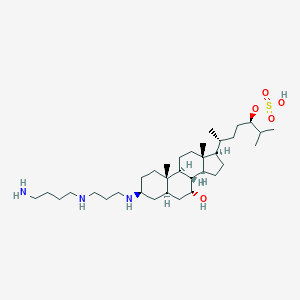
Squalamine
概要
説明
Squalamine is a naturally occurring cationic aminosterol first isolated from the liver of the dogfish shark (Squalus acanthias) . Structurally, it comprises a sulfated C27 bile acid conjugated to spermidine, rendering it amphiphilic and membrane-active . This unique architecture enables this compound to interact with negatively charged phospholipids in bacterial membranes, disrupting membrane integrity and inducing cell death via ATP depletion . Its broad-spectrum antimicrobial activity includes efficacy against Gram-positive (Staphylococcus aureus, MIC 3.12 µg/mL) and Gram-negative bacteria (Pseudomonas aeruginosa, MIC 8 µg/mL), fungi, and protozoa .
Beyond antimicrobial effects, this compound exhibits potent antiangiogenic properties by inhibiting vascular endothelial growth factor (VEGF)-induced signaling pathways in endothelial cells. It disrupts F-actin stress fibers, reduces VE-cadherin expression, and modulates intracellular pH via Na+/H+ exchanger (NHE3) inhibition, thereby curbing tumor-associated angiogenesis . Clinical trials highlight its synergy with platinum-based chemotherapies (e.g., cisplatin, carboplatin), enhancing tumor growth inhibition in lung, ovarian, and breast cancers .
準備方法
合成経路と反応条件: スクアラミンは、コール酸から始まる一連の化学反応によって合成できます。 このプロセスには、ステロイド骨格の修飾、スペルミジンフラグメントの取得、さまざまな保護基の脱保護、およびC24位のスルホン化が含まれます . 一例として、1,3-ジアミノプロパンをクロロブタノールでアルキル化し、アミノ基とアルコール基を保護した後、アジドに変換し、3-ケトコレステロールの24R-硫酸塩を還元アミノ化し、触媒的水素分解することでスクアラミンを得ます .
工業生産方法: スクアラミンの工業生産は、この化合物の最も豊富な供給源であるアイザメの肝臓からの抽出を含みます。 抽出プロセスは、スクアラミンや他のアミノステロールを大量に得られるように最適化されています .
化学反応の分析
反応の種類: スクアラミンは、以下のものを含むさまざまな化学反応を起こします。
酸化: スクアラミンは、酸化されてさまざまな誘導体を形成します。
還元: 還元反応は、スクアラミン分子の官能基を修飾できます。
置換: スクアラミンは、特にアミノ基とヒドロキシル基で置換反応を起こす可能性があります。
一般的な試薬と条件:
酸化: 一般的な酸化剤には、過マンガン酸カリウムと三酸化クロムが含まれます。
還元: 水素化リチウムアルミニウムや水素化ホウ素ナトリウムなどの還元剤が使用されます。
置換: 置換反応は、多くの場合、ハロアルカンやアシルクロリドなどの試薬を使用します。
主な生成物: これらの反応から生成される主な生成物には、官能基が修飾されたさまざまなスクアラミン誘導体が含まれ、これにより生物学的活性が向上したり変化したりすることがあります .
4. 科学研究への応用
スクアラミンは、さまざまな科学研究に幅広く応用されています。
化学: 他のアミノステロールやステロイド誘導体の合成の前駆体として使用されます。
生物学: 多剤耐性菌を含むさまざまな病原体に対する抗菌活性が研究されています.
科学的研究の応用
Squalamine has a wide range of scientific research applications:
Chemistry: Used as a precursor for synthesizing other aminosterols and steroid derivatives.
Medicine: Investigated for its antiangiogenic properties, making it a potential therapeutic agent for cancer and age-related macular degeneration.
作用機序
スクアラミンは、界面活性剤のようなメカニズムによってその効果を発揮します。 細菌膜の負電荷を帯びたリン酸基と相互作用し、膜の脱分極と破壊につながります . このメカニズムは、カチオン性ペプチド系抗生物質に似ており、膜に半安定な孔を形成することが関与しています .
類似化合物:
トロドスケミン: アイザメから単離された別のアミノステロールで、抗血管新生作用と神経保護作用で知られています.
セラゲニン: コール酸の合成誘導体で、抗菌活性は同様です.
クララミン: 比較可能な生物学的活性を有するトリテルペノイド.
スクアラミンの独自性: スクアラミンの独特の構造は、スペルミジンがC-27硫酸化胆汁酸に結合したものであり、他のアミノステロールとは異なります。 スクアラミンは、広範囲の抗菌活性とさまざまな分野における潜在的な治療応用を持つことから、科学研究においてその重要性を際立たせています .
類似化合物との比較
Squalamine belongs to a family of aminosterols with diverse biological activities. Below is a detailed comparison with structurally or functionally related compounds:
Trodusquemine
- Structure : A des-sulfated analog of this compound, retaining the spermidine conjugate but lacking the sulfate group .
- Mechanism : Shares antiangiogenic properties but exhibits broader antimicrobial activity, including against protozoa and multidrug-resistant bacteria .
- Higher efficacy against Candida albicans (MIC 4 µg/mL vs. This compound’s 8 µg/mL) .
- Therapeutic Applications : Explored for neurodegenerative diseases (e.g., Parkinson’s) due to its ability to inhibit α-synuclein aggregation .
Claramine
- Structure: Synthetic aminosterol with a modified polyamine chain .
- Mechanism : Targets bacterial membranes but with enhanced lipophilicity, improving activity against mycobacteria (MIC 5–25 µg/mL) .
- Key Differences :
Petromyzonamine Disulfate (PADS)
- Structure : Derived from this compound via primary amine oxidation and 7-OH sulfation, found in sea lamprey pheromones .
- Mechanism : Functions as a migratory pheromone but lacks direct antimicrobial activity .
- Key Differences: Biosynthetically related but diverges in function (chemical signaling vs. host defense). Highlights evolutionary repurposing of aminosterols across species .
Comparative Data Tables
Table 1: Antimicrobial Activity of this compound and Analogs
Table 2: Antiangiogenic and Anticancer Effects
Key Research Findings
Antimicrobial Selectivity : this compound’s preference for phosphatidylglycerol in bacterial membranes explains its low toxicity to eukaryotic cells . Its analogs (e.g., 223/224) achieve mycobacterial activity via increased lipophilicity but sacrifice broad-spectrum potency .
Antiangiogenic Synergy: this compound enhances platinum-based chemotherapy by normalizing tumor vasculature, reducing hypoxia, and increasing drug delivery . In contrast, trodusquemine’s focus on neurodegenerative pathways limits its oncological applications .
Clinical Safety : Phase I trials established this compound’s safety (MTD = 500 mg/m²/day) with reversible hepatotoxicity, while analogs remain in preclinical stages .
生物活性
Squalamine is a natural aminosterol derived from the tissues of the dogfish shark (Squalus acanthias). It has garnered attention for its diverse biological activities, particularly in the fields of oncology and microbiology. This article explores the biological activity of this compound, focusing on its mechanisms of action, therapeutic applications, and relevant clinical findings.
This compound exhibits multiple mechanisms that contribute to its biological activity:
- Antiangiogenic Activity : this compound inhibits the proliferation and migration of endothelial cells, which are crucial for angiogenesis. This effect is mediated through the inhibition of key signaling pathways involving vascular endothelial growth factor (VEGF), platelet-derived growth factor (PDGF), and basic fibroblast growth factor (bFGF) . this compound disrupts intracellular calmodulin, leading to altered cellular signaling that prevents neovascularization .
- Antitumor Effects : In various animal models, this compound has demonstrated significant antitumor activity. For instance, in a glioma xenograft model, this compound reduced tumor growth by 36-43% at a dose of 20 mg/kg/day . Additionally, when combined with chemotherapy agents like cyclophosphamide and cisplatin, this compound enhanced tumor response rates and delayed progression in breast cancer models .
- Antibacterial Properties : this compound has shown potent antibacterial activity against both Gram-negative and Gram-positive bacteria, including multidrug-resistant strains. Its mechanism involves disrupting bacterial membranes, leading to ATP release and cell death . This unique action positions this compound as a promising candidate in the fight against antibiotic resistance.
Antitumor Studies
Several clinical trials have investigated the efficacy of this compound in cancer treatment:
- Phase I Study : A study involving 19 patients assessed the pharmacokinetics and safety of continuous intravenous infusion of this compound. The maximum tolerated dose (MTD) was determined to be 192 mg/m²/day, with dose-limiting toxicities primarily related to hepatotoxicity . Partial tumor responses were observed in patients with various cancers.
- Phase I/IIA Trial : In this trial, this compound was administered alongside standard chemotherapy for non-small cell lung cancer. The results indicated a clinical response rate of 34% among evaluable patients, with a median survival of 10 months .
Antimicrobial Studies
This compound's antibacterial properties have been highlighted in studies focusing on its efficacy against resistant bacterial strains:
- A study demonstrated that this compound effectively disrupted the membranes of Staphylococcus aureus and Streptococcus pneumoniae, resulting in significant bacterial cell death .
Table: Summary of Clinical Trials Involving this compound
Study Type | Patient Population | Dosage | Outcome |
---|---|---|---|
Phase I Study | Advanced cancer patients | 192 mg/m²/day (MTD) | Partial responses; hepatotoxicity noted |
Phase I/IIA Trial | Non-small cell lung cancer patients | 300 mg/m²/day | 34% clinical response; median survival 10 months |
Antibacterial Study | Multidrug-resistant bacteria | Various concentrations | Effective against Gram-positive & Gram-negative bacteria |
Q & A
Basic Research Questions
Q. What experimental models are validated for studying Squalamine’s antitumor and anti-angiogenic effects?
Researchers commonly use:
- Human umbilical vein endothelial cells (HUVECs) for in vitro assays evaluating VEGF-induced proliferation and capillary tube formation .
- Xenograft models (e.g., MCF-7 breast cancer, lung, and glioblastoma tumors in rodents) to assess tumor growth suppression and angiogenesis inhibition .
- Bacterial membrane models (e.g., LPS monolayers) to study antimicrobial mechanisms . Methodological Note: Prioritize HER2-overexpressing cell lines to maximize VEGF-driven angiogenic responses .
Q. What molecular targets mediate this compound’s anti-angiogenic activity?
Key targets include:
- VEGF signaling : this compound blocks VEGF-induced endothelial proliferation by disrupting FAK phosphorylation and F-actin stress fiber organization in HUVECs .
- Integrins : Reduces endothelial adhesion and migration via integrin suppression .
- NHE3 exchanger : Inhibits brush-border Na+/H+ exchange, indirectly affecting cell volume and shape . Experimental Design: Combine Western blotting (for FAK phosphorylation) and confocal microscopy (for cytoskeletal changes) to validate mechanisms .
Q. How is this compound synthesized for research applications?
this compound is chemically synthesized via:
- Reductive amination reactions using sterol precursors and polyamines .
- Modifications to enhance bioavailability, such as lactate salt formulations . Quality Control: Verify purity using NMR and mass spectrometry, referencing the original 1993 structural characterization .
Q. What are the established safety and pharmacokinetic profiles of this compound in preclinical models?
- Safety : No cytotoxic effects observed in endothelial or cancer cells at therapeutic doses (≤3.2 μM) .
- Pharmacokinetics : Ineffective via intravitreal administration but shows systemic efficacy in rodents and humans at 2–4 mg/kg doses . Methodological Note: Use intravenous or intraperitoneal routes for systemic studies; monitor plasma stability using HPLC .
Q. How does this compound exert broad-spectrum antimicrobial activity?
Mechanism:
- Cationic amphipathic structure disrupts bacterial membranes (Gram-negative and -positive) by neutralizing LPS electrostatic charges .
- Minimal eukaryotic membrane interaction due to glycolipid sugar groups impairing insertion . Assay Design: Use ATP release assays and dye permeability tests to quantify membrane disruption .
Advanced Research Questions
Q. How can contradictory data on this compound’s efficacy across administration routes be reconciled?
Contradictions arise from:
- Tissue-specific bioavailability : Intravitreal dosing fails due to rapid clearance, while systemic dosing achieves therapeutic plasma levels .
- Tumor microenvironment : HER2-overexpressing tumors show enhanced sensitivity due to elevated VEGF secretion . Strategy: Conduct comparative pharmacokinetic studies across routes and tissues, using radiolabeled this compound .
Q. What methodologies optimize this compound concentrations for anti-angiogenesis assays?
Parameter | Optimal Range | Key Findings | Reference |
---|---|---|---|
HUVEC proliferation | 160 nM – 3.2 μM | Dose-dependent VEGF inhibition; IC₅₀ ~1 μM | |
Capillary tube formation | 1 μM | Complete disruption of VEGF-induced tubes | |
Bacterial membrane disruption | 0.5 mg/mL | LPS monolayer penetration | |
Experimental Tip: Perform dose-response curves with negative controls (e.g., VEGF-only) to isolate this compound-specific effects . |
Q. How can researchers differentiate this compound’s direct antitumor effects from its anti-angiogenic actions?
Approaches include:
- Co-culture systems : Separate tumor cells and endothelial cells to assess indirect angiogenesis suppression .
- VEGF knockout models : Use CRISPR-edited cancer cells to eliminate VEGF secretion, isolating direct effects .
- Metabolic profiling : Compare ATP levels in treated tumor vs. endothelial cells to identify cytotoxicity .
Q. What strategies improve this compound’s efficacy in combination therapies?
- Synergy with chemotherapy : Weekly “metronomic” dosing (100–200 mg/m²) with carboplatin/paclitaxel improves NSCLC response rates but not survival .
- HER2-targeted agents : Combine with trastuzumab for enhanced regression in HER2+ breast tumors .
- Antiviral cocktails : Pair with nucleoside analogs (e.g., ribavirin) to exploit membrane charge neutralization . Trial Design: Use adaptive randomization in phase II trials to identify optimal combinations .
Q. What novel mechanisms explain this compound’s antiviral activity beyond membrane charge neutralization?
Emerging hypotheses:
- Intracellular signaling modulation : Interaction with NHE3 may alter proton gradients critical for viral replication .
- Immune priming : Indirect activation of innate antiviral pathways via endothelial cell stabilization . Assay Recommendations: Use plaque reduction neutralization tests (PRNTs) and qPCR for viral load quantification .
特性
IUPAC Name |
[(3R,6R)-6-[(3S,5R,7R,8R,9S,10S,13R,14S,17R)-3-[3-(4-aminobutylamino)propylamino]-7-hydroxy-10,13-dimethyl-2,3,4,5,6,7,8,9,11,12,14,15,16,17-tetradecahydro-1H-cyclopenta[a]phenanthren-17-yl]-2-methylheptan-3-yl] hydrogen sulfate | |
---|---|---|
Source | PubChem | |
URL | https://pubchem.ncbi.nlm.nih.gov | |
Description | Data deposited in or computed by PubChem | |
InChI |
InChI=1S/C34H65N3O5S/c1-23(2)31(42-43(39,40)41)12-9-24(3)27-10-11-28-32-29(14-16-34(27,28)5)33(4)15-13-26(21-25(33)22-30(32)38)37-20-8-19-36-18-7-6-17-35/h23-32,36-38H,6-22,35H2,1-5H3,(H,39,40,41)/t24-,25-,26+,27-,28+,29+,30-,31-,32+,33+,34-/m1/s1 | |
Source | PubChem | |
URL | https://pubchem.ncbi.nlm.nih.gov | |
Description | Data deposited in or computed by PubChem | |
InChI Key |
UIRKNQLZZXALBI-MSVGPLKSSA-N | |
Source | PubChem | |
URL | https://pubchem.ncbi.nlm.nih.gov | |
Description | Data deposited in or computed by PubChem | |
Canonical SMILES |
CC(C)C(CCC(C)C1CCC2C1(CCC3C2C(CC4C3(CCC(C4)NCCCNCCCCN)C)O)C)OS(=O)(=O)O | |
Source | PubChem | |
URL | https://pubchem.ncbi.nlm.nih.gov | |
Description | Data deposited in or computed by PubChem | |
Isomeric SMILES |
C[C@H](CC[C@H](C(C)C)OS(=O)(=O)O)[C@H]1CC[C@@H]2[C@@]1(CC[C@H]3[C@H]2[C@@H](C[C@@H]4[C@@]3(CC[C@@H](C4)NCCCNCCCCN)C)O)C | |
Source | PubChem | |
URL | https://pubchem.ncbi.nlm.nih.gov | |
Description | Data deposited in or computed by PubChem | |
Molecular Formula |
C34H65N3O5S | |
Source | PubChem | |
URL | https://pubchem.ncbi.nlm.nih.gov | |
Description | Data deposited in or computed by PubChem | |
DSSTOX Substance ID |
DTXSID40869971 | |
Record name | Squalamine | |
Source | EPA DSSTox | |
URL | https://comptox.epa.gov/dashboard/DTXSID40869971 | |
Description | DSSTox provides a high quality public chemistry resource for supporting improved predictive toxicology. | |
Molecular Weight |
628.0 g/mol | |
Source | PubChem | |
URL | https://pubchem.ncbi.nlm.nih.gov | |
Description | Data deposited in or computed by PubChem | |
CAS No. |
148717-90-2 | |
Record name | Squalamine | |
Source | CAS Common Chemistry | |
URL | https://commonchemistry.cas.org/detail?cas_rn=148717-90-2 | |
Description | CAS Common Chemistry is an open community resource for accessing chemical information. Nearly 500,000 chemical substances from CAS REGISTRY cover areas of community interest, including common and frequently regulated chemicals, and those relevant to high school and undergraduate chemistry classes. This chemical information, curated by our expert scientists, is provided in alignment with our mission as a division of the American Chemical Society. | |
Explanation | The data from CAS Common Chemistry is provided under a CC-BY-NC 4.0 license, unless otherwise stated. | |
Record name | Squalamine [INN] | |
Source | ChemIDplus | |
URL | https://pubchem.ncbi.nlm.nih.gov/substance/?source=chemidplus&sourceid=0148717902 | |
Description | ChemIDplus is a free, web search system that provides access to the structure and nomenclature authority files used for the identification of chemical substances cited in National Library of Medicine (NLM) databases, including the TOXNET system. | |
Record name | Squalamine | |
Source | DrugBank | |
URL | https://www.drugbank.ca/drugs/DB06461 | |
Description | The DrugBank database is a unique bioinformatics and cheminformatics resource that combines detailed drug (i.e. chemical, pharmacological and pharmaceutical) data with comprehensive drug target (i.e. sequence, structure, and pathway) information. | |
Explanation | Creative Common's Attribution-NonCommercial 4.0 International License (http://creativecommons.org/licenses/by-nc/4.0/legalcode) | |
Record name | Squalamine | |
Source | EPA DSSTox | |
URL | https://comptox.epa.gov/dashboard/DTXSID40869971 | |
Description | DSSTox provides a high quality public chemistry resource for supporting improved predictive toxicology. | |
Record name | SQUALAMINE | |
Source | FDA Global Substance Registration System (GSRS) | |
URL | https://gsrs.ncats.nih.gov/ginas/app/beta/substances/F8PO54Z4V7 | |
Description | The FDA Global Substance Registration System (GSRS) enables the efficient and accurate exchange of information on what substances are in regulated products. Instead of relying on names, which vary across regulatory domains, countries, and regions, the GSRS knowledge base makes it possible for substances to be defined by standardized, scientific descriptions. | |
Explanation | Unless otherwise noted, the contents of the FDA website (www.fda.gov), both text and graphics, are not copyrighted. They are in the public domain and may be republished, reprinted and otherwise used freely by anyone without the need to obtain permission from FDA. Credit to the U.S. Food and Drug Administration as the source is appreciated but not required. | |
Retrosynthesis Analysis
AI-Powered Synthesis Planning: Our tool employs the Template_relevance Pistachio, Template_relevance Bkms_metabolic, Template_relevance Pistachio_ringbreaker, Template_relevance Reaxys, Template_relevance Reaxys_biocatalysis model, leveraging a vast database of chemical reactions to predict feasible synthetic routes.
One-Step Synthesis Focus: Specifically designed for one-step synthesis, it provides concise and direct routes for your target compounds, streamlining the synthesis process.
Accurate Predictions: Utilizing the extensive PISTACHIO, BKMS_METABOLIC, PISTACHIO_RINGBREAKER, REAXYS, REAXYS_BIOCATALYSIS database, our tool offers high-accuracy predictions, reflecting the latest in chemical research and data.
Strategy Settings
Precursor scoring | Relevance Heuristic |
---|---|
Min. plausibility | 0.01 |
Model | Template_relevance |
Template Set | Pistachio/Bkms_metabolic/Pistachio_ringbreaker/Reaxys/Reaxys_biocatalysis |
Top-N result to add to graph | 6 |
Feasible Synthetic Routes
試験管内研究製品の免責事項と情報
BenchChemで提示されるすべての記事および製品情報は、情報提供を目的としています。BenchChemで購入可能な製品は、生体外研究のために特別に設計されています。生体外研究は、ラテン語の "in glass" に由来し、生物体の外で行われる実験を指します。これらの製品は医薬品または薬として分類されておらず、FDAから任何の医療状態、病気、または疾患の予防、治療、または治癒のために承認されていません。これらの製品を人間または動物に体内に導入する形態は、法律により厳格に禁止されています。これらのガイドラインに従うことは、研究と実験において法的および倫理的な基準の遵守を確実にするために重要です。