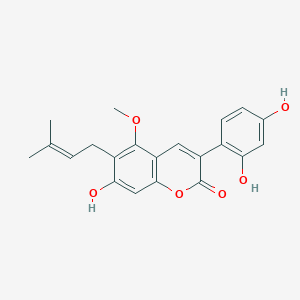
Glycycoumarin
説明
Glycycoumarin is a naturally occurring coumarin compound found in many plants and is known for its wide range of biological activities. It is a secondary metabolite of many plant species, including Glycyrrhiza glabra, Prunella vulgaris, and Angelica sinensis. It has been used as a traditional Chinese medicine for centuries and is now also used in modern medicine for its various pharmacological activities and therapeutic applications.
科学的研究の応用
Glycycoumarin: A Comprehensive Analysis of Scientific Research Applications
Anti-Hepatoma Effect: Glycycoumarin has demonstrated a potent anti-hepatoma effect in both cell culture and HepG2 xenograft models. It shows preventive effects on cancers induced by various human hepatoma cells, including HepG2, Huh7, and human prostate cancer DU-145 .
Liver Protection: In vitro models have shown that Glycycoumarin can significantly suppress apoptosis in HepG2 cells and prevent lipid accumulation induced by oleic acid/palmitic acid. In vivo, it reverses biochemical and pathological changes in diet-induced liver damage in mice .
Estrogen-Responsive Gene Expression Regulation: Glycycoumarin was found to be effective in increasing the expression of estrogen-responsive genes such as PgR and GREB1 in MCF7 breast cancer cells. It has intrinsic activity comparable to E2 ubiquitin-conjugating enzymes, stimulating proliferation with significant agonist activity .
Antispasmodic Activity: Studies have indicated that Glycycoumarin possesses antispasmodic properties, although specific details on this application were not provided in the search results.
Antibacterial and Antivirus Activities: Research has highlighted Glycycoumarin’s biological activities which include antibacterial and antivirus effects .
Antioxidant Properties: Glycycoumarin is reported to have antioxidant activities, contributing to its potential therapeutic applications .
Anti-Inflammatory Effects: Alongside its antioxidant properties, Glycycoumarin also exhibits anti-inflammatory activities .
作用機序
Target of Action
Glycycoumarin is a representative coumarin compound with significant pharmacological activities isolated from Glycyrrhiza uralensis Fisch., Fabaceae . It has been shown to have many biological activities, such as anti-tumor, liver protection, antispasmodic, antibacterial, and antivirus .
Mode of Action
It has been suggested that it can exert a good anti-hepatoma effect in cell culture and the hepg2 xenograft model . It has also been reported to degrade P62-mediated Keap1 by activating autophagy, thereby reducing alcohol-induced hepatotoxicity .
Biochemical Pathways
Glycycoumarin is known to affect several biochemical pathways. It has been reported to enhance the expression of the pro-apoptotic BCL2 family of proteins and decrease the expression of the anti-apoptotic BCL2 family . This could inhibit mitochondrial activation and regulate hepatic lipid metabolism .
Result of Action
The result of Glycycoumarin’s action is primarily seen in its hepatoprotective effects. It protects hepatocytes through activation of autophagy and mitigation of ER stress/GSK-3 –JNK/CHOP-mitochondria cascade activation .
Action Environment
The action of Glycycoumarin can be influenced by various environmental factors. For instance, due to hepatic and/or intestinal tract first-pass metabolism, Glycycoumarin exhibits a poor bioavailability of 9.22%, as estimated from its total plasma concentration . Additionally, only a small amount of Glycycoumarin is excreted via bile and urine .
特性
IUPAC Name |
3-(2,4-dihydroxyphenyl)-7-hydroxy-5-methoxy-6-(3-methylbut-2-enyl)chromen-2-one | |
---|---|---|
Source | PubChem | |
URL | https://pubchem.ncbi.nlm.nih.gov | |
Description | Data deposited in or computed by PubChem | |
InChI |
InChI=1S/C21H20O6/c1-11(2)4-6-14-18(24)10-19-16(20(14)26-3)9-15(21(25)27-19)13-7-5-12(22)8-17(13)23/h4-5,7-10,22-24H,6H2,1-3H3 | |
Source | PubChem | |
URL | https://pubchem.ncbi.nlm.nih.gov | |
Description | Data deposited in or computed by PubChem | |
InChI Key |
NZYSZZDSYIBYLC-UHFFFAOYSA-N | |
Source | PubChem | |
URL | https://pubchem.ncbi.nlm.nih.gov | |
Description | Data deposited in or computed by PubChem | |
Canonical SMILES |
CC(=CCC1=C(C2=C(C=C1O)OC(=O)C(=C2)C3=C(C=C(C=C3)O)O)OC)C | |
Source | PubChem | |
URL | https://pubchem.ncbi.nlm.nih.gov | |
Description | Data deposited in or computed by PubChem | |
Molecular Formula |
C21H20O6 | |
Source | PubChem | |
URL | https://pubchem.ncbi.nlm.nih.gov | |
Description | Data deposited in or computed by PubChem | |
DSSTOX Substance ID |
DTXSID20241630 | |
Record name | 3-(2,4-Dihydroxy-phenyl)-7-hydroxy-5-methoxy-6-(3-methyl-but-2-enyl)-1-benzopyran-2-one | |
Source | EPA DSSTox | |
URL | https://comptox.epa.gov/dashboard/DTXSID20241630 | |
Description | DSSTox provides a high quality public chemistry resource for supporting improved predictive toxicology. | |
Molecular Weight |
368.4 g/mol | |
Source | PubChem | |
URL | https://pubchem.ncbi.nlm.nih.gov | |
Description | Data deposited in or computed by PubChem | |
Physical Description |
Solid | |
Record name | Glycycoumarin | |
Source | Human Metabolome Database (HMDB) | |
URL | http://www.hmdb.ca/metabolites/HMDB0038225 | |
Description | The Human Metabolome Database (HMDB) is a freely available electronic database containing detailed information about small molecule metabolites found in the human body. | |
Explanation | HMDB is offered to the public as a freely available resource. Use and re-distribution of the data, in whole or in part, for commercial purposes requires explicit permission of the authors and explicit acknowledgment of the source material (HMDB) and the original publication (see the HMDB citing page). We ask that users who download significant portions of the database cite the HMDB paper in any resulting publications. | |
Product Name |
Glycycoumarin | |
CAS RN |
94805-82-0 | |
Record name | Glycycoumarin | |
Source | CAS Common Chemistry | |
URL | https://commonchemistry.cas.org/detail?cas_rn=94805-82-0 | |
Description | CAS Common Chemistry is an open community resource for accessing chemical information. Nearly 500,000 chemical substances from CAS REGISTRY cover areas of community interest, including common and frequently regulated chemicals, and those relevant to high school and undergraduate chemistry classes. This chemical information, curated by our expert scientists, is provided in alignment with our mission as a division of the American Chemical Society. | |
Explanation | The data from CAS Common Chemistry is provided under a CC-BY-NC 4.0 license, unless otherwise stated. | |
Record name | Glycycoumarin | |
Source | ChemIDplus | |
URL | https://pubchem.ncbi.nlm.nih.gov/substance/?source=chemidplus&sourceid=0094805820 | |
Description | ChemIDplus is a free, web search system that provides access to the structure and nomenclature authority files used for the identification of chemical substances cited in National Library of Medicine (NLM) databases, including the TOXNET system. | |
Record name | 3-(2,4-Dihydroxy-phenyl)-7-hydroxy-5-methoxy-6-(3-methyl-but-2-enyl)-1-benzopyran-2-one | |
Source | EPA DSSTox | |
URL | https://comptox.epa.gov/dashboard/DTXSID20241630 | |
Description | DSSTox provides a high quality public chemistry resource for supporting improved predictive toxicology. | |
Record name | GLYCYCOUMARIN | |
Source | FDA Global Substance Registration System (GSRS) | |
URL | https://gsrs.ncats.nih.gov/ginas/app/beta/substances/CWQ2B8G346 | |
Description | The FDA Global Substance Registration System (GSRS) enables the efficient and accurate exchange of information on what substances are in regulated products. Instead of relying on names, which vary across regulatory domains, countries, and regions, the GSRS knowledge base makes it possible for substances to be defined by standardized, scientific descriptions. | |
Explanation | Unless otherwise noted, the contents of the FDA website (www.fda.gov), both text and graphics, are not copyrighted. They are in the public domain and may be republished, reprinted and otherwise used freely by anyone without the need to obtain permission from FDA. Credit to the U.S. Food and Drug Administration as the source is appreciated but not required. | |
Record name | Glycycoumarin | |
Source | Human Metabolome Database (HMDB) | |
URL | http://www.hmdb.ca/metabolites/HMDB0038225 | |
Description | The Human Metabolome Database (HMDB) is a freely available electronic database containing detailed information about small molecule metabolites found in the human body. | |
Explanation | HMDB is offered to the public as a freely available resource. Use and re-distribution of the data, in whole or in part, for commercial purposes requires explicit permission of the authors and explicit acknowledgment of the source material (HMDB) and the original publication (see the HMDB citing page). We ask that users who download significant portions of the database cite the HMDB paper in any resulting publications. | |
Melting Point |
243.5 - 244.5 °C | |
Record name | Glycycoumarin | |
Source | Human Metabolome Database (HMDB) | |
URL | http://www.hmdb.ca/metabolites/HMDB0038225 | |
Description | The Human Metabolome Database (HMDB) is a freely available electronic database containing detailed information about small molecule metabolites found in the human body. | |
Explanation | HMDB is offered to the public as a freely available resource. Use and re-distribution of the data, in whole or in part, for commercial purposes requires explicit permission of the authors and explicit acknowledgment of the source material (HMDB) and the original publication (see the HMDB citing page). We ask that users who download significant portions of the database cite the HMDB paper in any resulting publications. | |
Retrosynthesis Analysis
AI-Powered Synthesis Planning: Our tool employs the Template_relevance Pistachio, Template_relevance Bkms_metabolic, Template_relevance Pistachio_ringbreaker, Template_relevance Reaxys, Template_relevance Reaxys_biocatalysis model, leveraging a vast database of chemical reactions to predict feasible synthetic routes.
One-Step Synthesis Focus: Specifically designed for one-step synthesis, it provides concise and direct routes for your target compounds, streamlining the synthesis process.
Accurate Predictions: Utilizing the extensive PISTACHIO, BKMS_METABOLIC, PISTACHIO_RINGBREAKER, REAXYS, REAXYS_BIOCATALYSIS database, our tool offers high-accuracy predictions, reflecting the latest in chemical research and data.
Strategy Settings
Precursor scoring | Relevance Heuristic |
---|---|
Min. plausibility | 0.01 |
Model | Template_relevance |
Template Set | Pistachio/Bkms_metabolic/Pistachio_ringbreaker/Reaxys/Reaxys_biocatalysis |
Top-N result to add to graph | 6 |
Feasible Synthetic Routes
Q & A
Q1: How does GCM exert its anti-cancer activity?
A1: Research indicates GCM demonstrates potent anti-liver cancer activity by directly targeting and inactivating the oncogenic kinase T-LAK cell-originated protein kinase (TOPK) [[6]]. This inactivation leads to the activation of the p53 pathway, inducing apoptosis and inhibiting tumor growth [[6], []].
Q2: What is the role of GCM in protecting against acetaminophen-induced liver injury?
A2: GCM exhibits hepatoprotective effects against acetaminophen-induced liver injury, primarily by activating sustained autophagy, which aids in cellular repair and protection [[10], []].
Q3: Does GCM play a role in modulating ER stress?
A3: Yes, GCM has shown a protective effect against acetaminophen overdose in mice by enhancing TEX264-mediated ER-phagy, a selective autophagy of the endoplasmic reticulum, which helps to alleviate ER stress and promote liver regeneration [[9]].
Q4: How does GCM contribute to the anti-diabetic potential of licorice?
A4: GCM, along with other compounds in licorice, exhibits anti-diabetic effects. It has been shown to act as an α-glucosidase inhibitor, potentially aiding in blood glucose control [[13]].
Q5: What is the mechanism behind GCM's antispasmodic activity?
A5: Research suggests that GCM contributes to the antispasmodic properties of licorice. It has been shown to suppress colonic peristalsis, potentially by inhibiting phosphodiesterase 3 [[2], [], []].
Q6: What is the molecular formula and weight of GCM?
A6: The molecular formula of GCM is C20H18O4 and its molecular weight is 322.35 g/mol.
Q7: Are there any spectroscopic data available for GCM?
A7: Spectroscopic data, including ESI-MS, ESI-MSn, and ultraviolet (UV) spectra, have been utilized for the identification of GCM in Glycyrrhiza uralensis extracts [[16]].
Q8: Does GCM exhibit any catalytic properties?
A8: The provided research primarily focuses on the pharmacological activities of GCM and does not delve into its catalytic properties.
Q9: Have computational methods been employed to study GCM?
A10: Yes, molecular docking simulations have been employed to investigate the interactions between GCM and specific targets, such as PXR and Keap1, providing insights into its potential binding mechanisms [[18], []].
Q10: How do structural modifications of GCM affect its activity?
A11: While specific SAR studies for GCM are not extensively discussed in the provided research, the presence of prenyl groups in GCM and other licorice compounds is highlighted for their potential contribution to antibacterial activity [[13], [], []].
Q11: Are there any specific formulation strategies mentioned to improve the stability, solubility, or bioavailability of GCM?
A11: The provided research primarily focuses on identifying and characterizing GCM within licorice and does not discuss specific formulation strategies for this compound.
Q12: Is there any information available on the SHE regulations, compliance, risk minimization, and responsible practices associated with GCM?
A12: Specific SHE regulations related to GCM are not discussed in the provided research articles.
Q13: What is known about the absorption, distribution, metabolism, and excretion of GCM?
A14: Studies in rats indicate that GCM is rapidly absorbed after oral administration, extensively metabolized primarily via glucuronidation, and distributed widely in tissues except for the brain [[7], []]. It exhibits low bioavailability due to significant first-pass metabolism [[7]].
Q14: What is the duration of action of GCM after oral administration?
A14: Specific information regarding the duration of action of GCM is not available in the provided research.
Q15: What types of in vitro and in vivo models have been used to study the effects of GCM?
A16: Various in vitro models, including human liver cancer cell lines (HepG2, Huh-7, and SMMC-7721), and in vivo models, including mouse models of liver injury and diabetic KK-Ay mice, have been utilized to investigate the effects of GCM [[6], [], [], []].
Q16: Are there any known resistance mechanisms associated with GCM?
A16: While the research does not directly address GCM resistance, one study highlights the potential role of de novo lipogenesis and the TOPK-survivin axis in mediating resistance to ABT-737, a BH3 mimetic, in liver cancer cells. GCM was found to sensitize these cells to ABT-737 by targeting these resistance mechanisms [[12]].
Q17: Is there any information available on the toxicity and safety profile of GCM?
A17: While the research primarily focuses on the pharmacological benefits of GCM, one study utilizing a 3D-intestinal organoid model suggests that GCM might exhibit cytotoxicity at higher concentrations, emphasizing the need for further safety evaluations [[25]].
Q18: Are there any specific drug delivery and targeting strategies being explored for GCM?
A18: The provided research does not delve into specific drug delivery and targeting strategies for GCM.
Q19: What analytical methods have been used to characterize and quantify GCM?
A21: Various analytical techniques, including high-performance liquid chromatography (HPLC) coupled with mass spectrometry (MS) and ultra-high-performance liquid chromatography (UHPLC) coupled with triple-quadrupole mass spectrometry (QQQMS), have been employed to identify and quantify GCM in licorice samples [[5], [], []].
Q20: Is there any information available regarding the environmental impact and degradation of GCM?
A20: The provided research does not address the environmental impact and degradation of GCM.
Q21: Are there any studies on the dissolution rate and solubility of GCM in various media?
A21: Specific information regarding the dissolution rate and solubility of GCM is not available in the provided research.
Q22: Have the analytical methods used to quantify GCM been validated?
A24: While specific validation details are not extensively described, studies employing analytical techniques for GCM quantification mention parameters such as linearity, recovery rates, and relative standard deviations, suggesting method validation procedures [[5], []].
Q23: What measures are taken to ensure the quality and consistency of GCM?
A23: The research highlights the importance of chemical standardization for licorice due to the variations in active compound content among different species. Techniques like UHPLC-MS/MS are suggested for accurate identification and quantification of GCM and other constituents in licorice raw materials and dietary supplements, ensuring quality and consistency [[26]].
Q24: Does GCM elicit any immunological responses?
A24: Specific information regarding the immunogenicity and immunological responses associated with GCM is not provided in the research articles.
Q25: Are there any known interactions between GCM and drug transporters?
A25: The provided research does not explore potential interactions between GCM and drug transporters.
Q26: Can GCM affect drug-metabolizing enzymes?
A26: Research suggests that licorice and its constituents, including GCM, can potentially affect the activity of drug-metabolizing enzymes, particularly CYP3A4 and CYP1A2, which could influence the metabolism and clearance of certain drugs [[1]].
Q27: What is known about the biocompatibility and biodegradability of GCM?
A27: The provided research focuses on the pharmacological effects of GCM and does not provide specific details regarding its biocompatibility and biodegradability.
Q28: Are there any strategies for recycling GCM or managing waste generated during its production or use?
A28: The research primarily focuses on the pharmaceutical aspects of GCM and does not delve into its recycling or waste management strategies.
Q29: What research infrastructure and resources are available for studying GCM?
A32: The research highlights the use of advanced analytical techniques such as UHPLC-MS/MS, indicating the availability of sophisticated infrastructure and resources for studying GCM and other licorice constituents [[1], []].
Q30: What is the historical context of GCM research?
A33: While specific historical milestones are not explicitly outlined, the research acknowledges licorice's long-standing use in traditional medicine, implying historical significance and a foundation for modern research on its constituents like GCM [[1], []].
Q31: Are there any cross-disciplinary applications or synergies involving GCM research?
A34: The research showcases the integration of disciplines like pharmacology, analytical chemistry, and computational modeling in studying GCM, highlighting cross-disciplinary efforts in understanding its properties and applications [[1], [], []].
試験管内研究製品の免責事項と情報
BenchChemで提示されるすべての記事および製品情報は、情報提供を目的としています。BenchChemで購入可能な製品は、生体外研究のために特別に設計されています。生体外研究は、ラテン語の "in glass" に由来し、生物体の外で行われる実験を指します。これらの製品は医薬品または薬として分類されておらず、FDAから任何の医療状態、病気、または疾患の予防、治療、または治癒のために承認されていません。これらの製品を人間または動物に体内に導入する形態は、法律により厳格に禁止されています。これらのガイドラインに従うことは、研究と実験において法的および倫理的な基準の遵守を確実にするために重要です。