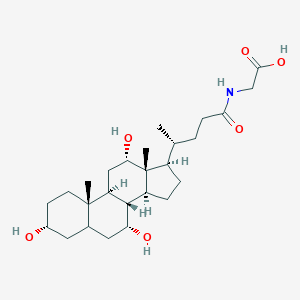
Glycocholic acid
概要
説明
Glycocholic acid (GCA) is a glycine-conjugated primary bile acid synthesized in the liver from cholic acid (CA). Its structure comprises a sterol ring system common to all bile acids, with a glycine moiety conjugated to the side chain at carbon 24 . This conjugation enhances its water solubility, facilitating emulsification of dietary lipids in the intestine. Clinically, GCA serves as a biomarker for liver dysfunction, with elevated serum levels correlating with disease severity, particularly in cirrhosis and chronic active hepatitis . Its role extends to immune modulation, as GCA enhances IgG/IgM production, CD4+ T-cell ratios, and cytokine levels in murine models .
準備方法
Chemical Synthesis of Glycocholic Acid
Mixed Anhydride Method
The most widely documented synthesis of GCA involves a three-step process: (1) mixed anhydride formation from cholic acid, (2) aminolysis with glycine esters, and (3) hydrolysis to yield the final product .
Mixed Anhydride Formation
Cholic acid is suspended in acetone or aqueous acetone with a trialkylamine base (e.g., triethylamine) at 18–25°C. Ethyl chloroformate is then added at 0–5°C to form the mixed anhydride intermediate. This step achieves a 76.5% yield (dry weight) after filtration .
Key Parameters:
-
Solvent: Acetone/water (50/50 v/v)
-
Base: Triethylamine (optimal for minimizing side reactions)
-
Temperature: 0–5°C during chloroformate addition
Aminolysis with Glycine Esters
The mixed anhydride reacts with glycine ethyl ester in aqueous solution under stirring for 12 hours. Ethyl acetate washes (35–40°C) remove unreacted intermediates, followed by vacuum distillation to eliminate residual solvents .
Reaction Efficiency:
Hydrolysis and Precipitation
The this compound ester undergoes alkaline hydrolysis (NaOH/KOH) to form the sodium salt, which is acidified to pH 2.0–2.5 with HCl. Precipitation yields GCA with a 92.3% stoichiometric efficiency .
Optimization of Reaction Conditions
Solvent Selection
Traditional methods used dimethylformamide (DMF) or dioxane, but acetone/water mixtures reduce side reactions and improve solubility of cholic acid .
Comparative Solvent Performance:
Solvent System | Reaction Yield | Purity |
---|---|---|
Acetone/water | 92.3% | >99% |
DMF | 85.1% | 95% |
Dioxane | 82.4% | 93% |
Temperature Control
Cooling during chloroformate addition (−5°C to +10°C) prevents thermal degradation of the mixed anhydride. Elevated temperatures during ethyl acetate washes (35–40°C) enhance impurity removal .
Purification and Isolation Techniques
Solid-Phase Extraction (SPE)
Post-synthesis purification employs Oasis MAX cartridges to isolate GCA from biological matrices .
SPE Protocol:
-
Conditioning: Methanol (1 mL) → water (1 mL)
-
Loading: Supernatant from protein precipitation
-
Washing: 5% NH₄OH (1 mL) → methanol (1 mL)
-
Elution: Methanol with 2% formic acid
Performance Metrics:
Analytical Validation of Synthesis
Chromatographic Separation
ID-LC–MS/MS with a 9-minute gradient elution resolves GCA from structural analogues (e.g., taurocholic acid, glycochenodeoxycholic acid) .
Chromatographic Conditions:
Parameter | Value |
---|---|
Column | C18 (2.1 × 100 mm) |
Flow Rate | 0.3 mL/min |
Gradient | 25–95% acetonitrile |
Precision and Accuracy
Metric | Intra-Day (%) | Inter-Day (%) |
---|---|---|
Precision | ≤1.30 | ≤1.80 |
Accuracy | 98.5–101.2 | 97.8–102.1 |
Challenges in GCA Preparation
Interference from Analogues
Endogenous disruptors in hepatobiliary disease samples co-elute with GCA under suboptimal LC conditions, causing overestimations of up to 20.3% .
Scalability Limitations
Industrial-scale synthesis faces challenges in maintaining cryogenic conditions during mixed anhydride formation, necessitating specialized reactor designs .
化学反応の分析
Table 1: Key Synthetic Methods for Glycocholic Acid
-
One-Pot Method : Combines condensation and hydrolysis steps in a single vessel, minimizing intermediate isolation. Wang et al. achieved >90% yield using NaOH for hydrolysis .
-
Mixed Anhydride Approach : Replaces toxic ethyl chloroformate with isobutyl chloroacetate, enhancing safety while maintaining efficiency .
Hydrolysis Reactions
Alkaline hydrolysis is pivotal in releasing this compound from ester derivatives:
Table 2: Hydrolysis Conditions and Outcomes
-
Hydrolysis under alkaline conditions cleaves ester bonds, yielding this compound or its sodium salt. Subsequent acidification precipitates the free acid .
In Vivo Biochemical Reactions
This compound undergoes enterohepatic circulation and enzymatic transformations:
-
Biosynthesis : Synthesized in the liver via conjugation of cholic acid with glycine. Enzymes like bile acid-CoA:amino acid N-acyltransferase (BAAT) catalyze this process .
-
Enterohepatic Recycling :
Anti-Inflammatory Mechanisms
This compound modulates inflammatory pathways through biochemical interactions:
Table 3: Pharmacological Reactivity in Inflammation
-
Mechanistic Insight : this compound binds farnesoid X receptor (FXR), suppressing pro-inflammatory cytokines and enhancing bile acid homeostasis .
Stability and Decomposition
科学的研究の応用
Glycocholate has numerous scientific research applications, including:
作用機序
グリココール酸は、脂肪の吸収を促進する界面活性剤として作用します。 食餌性脂肪と相互作用し、それを腸粘膜に容易に吸収される小さなミセルに分解します . 分子標的には食事中の脂質分子が含まれ、関与する経路は脂質消化と吸収に関連するものです .
類似化合物:
タウロコール酸: グリシンではなくタウリンと結合した別の胆汁酸結合体です。
コール酸: コール酸の非抱合型です。
デオキシコール酸: 腸内の細菌の作用によって、コール酸から生成される二次胆汁酸です.
比較: グリココール酸は、グリシン結合により、タウロコール酸やデオキシコール酸と比較して、溶解性が高く、代謝経路が異なるなどの特定の特性を付与されています . 脂肪の乳化における界面活性剤としての役割は、他の胆汁酸と同様ですが、特定の相互作用と吸収特性は異なっています .
類似化合物との比較
Comparison with Structurally Similar Bile Acids
Structural and Conformational Differences
GCA shares the sterol core with unconjugated cholic acid (CA) but differs in its glycine-conjugated side chain. Ion mobility–mass spectrometry (IM-MS) reveals that GCA’s collision cross-section (DTCCSN2) deviates from the mass-to-charge (m/z) trend observed in unconjugated bile acids. Despite a 57 Da mass increase from glycine conjugation, GCA’s DTCCSN2 values align with CA due to intramolecular hydrogen bonding between the glycine tail and the hydroxyl group at carbon 3, stabilizing its conformation . In contrast, taurocholic acid (TCA), conjugated with taurine, exhibits distinct side-chain interactions that may influence its spatial configuration (Figure 4 in ).
Table 1: Structural and Conformational Properties
Property | Glycocholic Acid (GCA) | Cholic Acid (CA) | Taurocholic Acid (TCA) |
---|---|---|---|
Conjugation | Glycine | None | Taurine |
Molecular Weight (Da) | 465.6 | 408.6 | 515.7 |
DTCCSN2 (Ų) | ~1 Ų increase vs. CA | Baseline | Not reported |
Key Interaction | C3-OH + glycine tail | C3-OH + tail | Sulfonic acid group |
Biochemical and Receptor Interactions
Enzyme Binding and Catalytic Activity
GCA exhibits stronger binding to bile salt hydrolase (BSH) from Lactobacillus plantarum compared to TCA, with a lower binding energy (-7.03 vs. -4.42 KJ/mol) and inhibition constant (252.24 µM vs. higher for TCA). Lys32 and Gly10 residues mediate hydrogen bonding with GCA, enhancing substrate-enzyme affinity .
Receptor Activation
- TGR5 Agonism : GCA is a weaker TGR5 agonist than taurolithocholic acid (TLCA) but stronger than unconjugated CA. Its EC50 for TGR5 activation is ~7-fold higher than CDCA-based conjugates (e.g., GCDCA) .
- Bitter Taste Receptors (TAS2R14) : GCA and l-Trp-Trp-Trp share pharmacophore similarities, enabling overlapping activation of TAS2R13. Mutagenesis studies show differential receptor responses, suggesting distinct binding modes compared to other agonists .
Table 2: Biochemical Interactions
Parameter | This compound (GCA) | Taurocholic Acid (TCA) | Chenodeoxycholic Acid (CDCA) |
---|---|---|---|
BSH Binding Energy (kJ/mol) | -7.03 | -4.42 | Not reported |
TGR5 EC50 | ~7-fold higher than CDCA conjugates | ~7-fold higher than CDCA conjugates | Lower than CA-based conjugates |
NTCP Transport Contribution (Na+-dependent) | 80% | Not reported | 40% (cholic acid) |
Physicochemical and Functional Differences
Liposome Interactions
In liposomal formulations, GCA reduces particle size more effectively than TCA at 0.5% and 1% concentrations. At 2%, both conjugates yield similar sizes, highlighting concentration-dependent effects on lipid bilayer dynamics .
Fiber Binding
GCA binds to dietary fibers (e.g., alfalfa, bran) with intermediate affinity compared to other bile salts. Its glycine moiety may reduce hydrophobic interactions, altering adsorption kinetics .
Clinical and Diagnostic Relevance
Disease Biomarkers
- Liver Disease : Elevated fasting serum GCA correlates with cirrhosis (86.6% sensitivity) but is less predictive than albumin or prothrombin activity .
- Pancreatic Cancer: GCA shows lower diagnostic sensitivity (AUC < 0.7) compared to creatine and inosine .
- Diabetic Kidney Disease (DKD) : Serum GCA inversely correlates with eGFR, suggesting renal excretion modulation .
Table 3: Clinical Performance of GCA vs. Other Biomarkers
Q & A
Q. What are the current methodologies for synthesizing glycocholic acid, and what are their limitations?
Basic Research Question
this compound is synthesized via enzymatic conjugation of cholic acid with glycine, primarily in the liver. Current methodologies include in vitro enzymatic synthesis using bile acid-CoA:amino acid N-acyltransferase (BAAT) and chemical synthesis via carbodiimide-mediated coupling. However, enzymatic methods face challenges in scalability due to enzyme instability, while chemical synthesis often yields impurities requiring rigorous purification (e.g., HPLC or column chromatography) . Recent advances propose microbial biosynthesis using engineered E. coli, but yields remain suboptimal for industrial applications .
Q. How can this compound be quantitatively detected in biological samples?
Basic Research Question
Colorimetric assays using nitrobenzene derivatives (e.g., 3,5-dinitrobenzoic acid) are standard for quantifying glycine-conjugated bile acids like this compound. The method relies on measuring absorbance at 540 nm, with a linear range of 12.5–200 nmol and molar absorption coefficients of ~1.56 × 10⁴ M⁻¹cm⁻¹ . For higher sensitivity, LC-MS/MS with MRM (multiple reaction monitoring) is recommended, achieving detection limits of 0.1 ng/mL in serum . Validate assays using spiked recovery experiments (85–115% recovery) to account for matrix effects.
Q. What molecular networking approaches are used to identify this compound derivatives in metabolomic studies?
Advanced Research Question
Global Natural Products Social Molecular Networking (GNPS) platforms enable annotation of this compound derivatives via mass shifts. For example, a parent ion at m/z 466.316 (this compound) can be linked to conjugates like m/z 556.363 (+90.047 Da, phenylalanine adduct) using Cytoscape visualization . Manual propagation of annotations requires verifying mass differences against theoretical adducts (e.g., +106.042 Da for tyrosine). Pair with MS² spectral matching to reference libraries (e.g., NIST) to confirm structural assignments .
Q. How does this compound function as a biomarker in liver diseases, and what are the challenges in validating its diagnostic utility?
Advanced Research Question
In acute hepatitis E-induced liver failure (HEV-ALF), this compound serum levels correlate with prognosis (AUC = 0.89 in ROC analysis). However, validation requires addressing confounding factors:
- Inter-individual variability : Bile acid profiles differ by diet, gut microbiota, and genetic polymorphisms (e.g., BAAT mutations).
- Pre-analytical variables : Fasting vs. postprandial sampling impacts concentrations. Standardize protocols to collect fasting samples .
- Analytical specificity : Differentiate this compound from isomers (e.g., glycochenodeoxycholate) using UPLC with C18 columns and 15-minute gradients .
Q. What are the proposed mechanisms by which this compound exhibits antitumor activity, and what experimental models support these findings?
Advanced Research Question
In murine breast cancer models (4T1 cells), this compound at 50 µM suppresses tumor growth by upregulating pro-apoptotic proteins (e.g., Bax) and inhibiting PI3K/Akt pathways. Western blot and RNA-seq data show dose-dependent increases in caspase-3 cleavage (1.5–2.5-fold) and reductions in cyclin D1 expression . Validate mechanisms using siRNA knockdown of bile acid receptors (e.g., TGR5) to confirm target specificity.
Q. How can researchers address contradictions in the reported pharmacological effects of this compound across different studies?
Advanced Research Question
Contradictions (e.g., pro- vs. anti-inflammatory effects) often arise from model-specific factors:
- Cell type variability : Primary hepatocytes vs. cancer cells may respond differently due to receptor expression levels.
- Concentration thresholds : Biphasic effects are observed (e.g., anti-inflammatory at 10 µM but cytotoxic at 100 µM). Conduct dose-response studies across 3–5 log scales .
- Meta-analysis : Use PRISMA guidelines to aggregate data from PubMed/Web of Science, applying random-effects models to quantify heterogeneity (I² statistic) .
Q. What are the key physicochemical properties of this compound that influence its role as a pharmaceutical excipient?
Basic Research Question
this compound’s amphiphilic structure (critical micellar concentration = 1.2 mM) enhances drug solubility via micelle formation. Its logP value (-1.5) and pKa (~4.5 for glycine conjugate) dictate pH-dependent stability in formulations. Use dynamic light scattering (DLS) to monitor micelle size (10–50 nm) and stability under accelerated storage conditions (40°C/75% RH) .
Q. What advanced chromatographic techniques are recommended for resolving this compound isomers in complex matrices?
Advanced Research Question
Ultra-performance convergence chromatography (UPCC) with supercritical CO₂ as mobile phase achieves baseline separation of this compound from taurocholic acid (α = 1.8) in bile. Optimize with 10 mM ammonium acetate in methanol co-solvent and a HSS C18 SB column (2.1 × 100 mm, 1.8 µm). For isomeric resolution (e.g., 3α vs. 3β hydroxyl), employ chiral columns (e.g., Chiralpak IA-3) with ethanol/hexane gradients .
Q. What are the standard protocols for ensuring the purity of this compound in experimental preparations?
Basic Research Question
Validate purity (>98%) via:
- TLC : Silica gel plates with chloroform/methanol/water (65:25:4), Rf = 0.35.
- HPLC : C18 column, 0.1% formic acid/acetonitrile gradient, retention time = 8.2 min.
- NMR : Confirm absence of tauro-conjugates via ¹H-NMR (δ 3.85 ppm for glycine methylene) . Store at -20°C under argon to prevent oxidation.
Q. How do bile acid conjugation patterns, including this compound, vary under pathological conditions, and what analytical strategies are used to study these variations?
Advanced Research Question
In prostate cancer (PCa), urinary this compound increases 2.3-fold due to dysregulated hepatic synthesis. Use OPLS-DA models to distinguish PCa (VIP scores >1.5) and validate with permutation testing (p < 0.01). For pathway analysis, KEGG enrichment of "primary bile acid biosynthesis" (p = 2.3 × 10⁻⁵) links this compound to disease progression. Combine FPLC/MS metabolomics with stable isotope tracing (¹³C-glycine) to quantify conjugation rates in vivo .
特性
CAS番号 |
475-31-0 |
---|---|
分子式 |
C26H43NO6 |
分子量 |
465.6 g/mol |
IUPAC名 |
2-[4-[(10S,13R)-3,7,12-trihydroxy-10,13-dimethyl-2,3,4,5,6,7,8,9,11,12,14,15,16,17-tetradecahydro-1H-cyclopenta[a]phenanthren-17-yl]pentanoylamino]acetic acid |
InChI |
InChI=1S/C26H43NO6/c1-14(4-7-22(31)27-13-23(32)33)17-5-6-18-24-19(12-21(30)26(17,18)3)25(2)9-8-16(28)10-15(25)11-20(24)29/h14-21,24,28-30H,4-13H2,1-3H3,(H,27,31)(H,32,33)/t14?,15?,16?,17?,18?,19?,20?,21?,24?,25-,26+/m0/s1 |
InChIキー |
RFDAIACWWDREDC-DPTHIFDSSA-N |
SMILES |
CC(CCC(=O)NCC(=O)O)C1CCC2C1(C(CC3C2C(CC4C3(CCC(C4)O)C)O)O)C |
異性体SMILES |
CC(CCC(=O)NCC(=O)O)C1CCC2[C@@]1(C(CC3C2C(CC4[C@@]3(CCC(C4)O)C)O)O)C |
正規SMILES |
CC(CCC(=O)NCC(=O)O)C1CCC2C1(C(CC3C2C(CC4C3(CCC(C4)O)C)O)O)C |
melting_point |
170 °C |
Key on ui other cas no. |
475-31-0 |
物理的記述 |
Dihydrate: Solid; [Sigma-Aldrich MSDS] |
溶解性 |
0.0033 mg/mL |
同義語 |
Cholylglycine Glycine Cholate Glycocholate Glycocholate Sodium Glycocholic Acid Glycocholic Acid, Sodium Salt |
製品の起源 |
United States |
Retrosynthesis Analysis
AI-Powered Synthesis Planning: Our tool employs the Template_relevance Pistachio, Template_relevance Bkms_metabolic, Template_relevance Pistachio_ringbreaker, Template_relevance Reaxys, Template_relevance Reaxys_biocatalysis model, leveraging a vast database of chemical reactions to predict feasible synthetic routes.
One-Step Synthesis Focus: Specifically designed for one-step synthesis, it provides concise and direct routes for your target compounds, streamlining the synthesis process.
Accurate Predictions: Utilizing the extensive PISTACHIO, BKMS_METABOLIC, PISTACHIO_RINGBREAKER, REAXYS, REAXYS_BIOCATALYSIS database, our tool offers high-accuracy predictions, reflecting the latest in chemical research and data.
Strategy Settings
Precursor scoring | Relevance Heuristic |
---|---|
Min. plausibility | 0.01 |
Model | Template_relevance |
Template Set | Pistachio/Bkms_metabolic/Pistachio_ringbreaker/Reaxys/Reaxys_biocatalysis |
Top-N result to add to graph | 6 |
Feasible Synthetic Routes
試験管内研究製品の免責事項と情報
BenchChemで提示されるすべての記事および製品情報は、情報提供を目的としています。BenchChemで購入可能な製品は、生体外研究のために特別に設計されています。生体外研究は、ラテン語の "in glass" に由来し、生物体の外で行われる実験を指します。これらの製品は医薬品または薬として分類されておらず、FDAから任何の医療状態、病気、または疾患の予防、治療、または治癒のために承認されていません。これらの製品を人間または動物に体内に導入する形態は、法律により厳格に禁止されています。これらのガイドラインに従うことは、研究と実験において法的および倫理的な基準の遵守を確実にするために重要です。