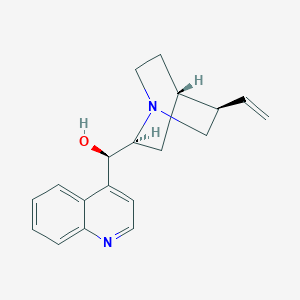
シンコニジン
概要
説明
Cinchonidine is an alkaloid found in the bark of most varieties of cinchona shrubs. It is a stereoisomer of cinchonine and has been historically significant in the synthesis of asymmetric organic compounds. Cinchonidine has been used in various medicinal applications, including the treatment of rheumatism, neuralgia, and sciatica .
科学的研究の応用
Cinchonidine has a wide range of applications in scientific research:
Chemistry: Used as a chiral ligand in asymmetric synthesis and as a resolving agent for racemic mixtures.
Biology: Studied for its potential antimalarial and antimicrobial properties.
Medicine: Investigated for its therapeutic effects in treating rheumatism, neuralgia, and sciatica.
Industry: Utilized in the production of chiral stationary phases for enantioselective chromatography
作用機序
Target of Action
Cinchonidine, a major member of the Cinchona alkaloids, is primarily used as an antimalarial agent . Its primary targets are the malaria-causing Plasmodium parasites . These parasites are responsible for the degradation of hemoglobin in erythrocytes .
Mode of Action
It is generally assumed that cinchonidine, like other cinchona alkaloids, prevents the polymerization of the toxic hematin (aquafe(iii)protoporphyrin ix) formed by the degradation of hemoglobin in erythrocytes to hemozoin (β-hematin) . This interaction inhibits the growth and multiplication of the Plasmodium parasites .
Biochemical Pathways
The biochemical pathways affected by Cinchonidine are related to the lifecycle of the Plasmodium parasites. By preventing the polymerization of toxic hematin, Cinchonidine disrupts the parasites’ ability to grow and multiply within red blood cells . This disruption affects the downstream effects of the parasites’ lifecycle, including their ability to cause the symptoms associated with malaria .
Pharmacokinetics
It has been reported that the mean plasma concentration for cinchonidine is approximately 25 mg/L . This suggests that Cinchonidine has a significant bioavailability, which is crucial for its effectiveness as an antimalarial agent .
Result of Action
The molecular and cellular effects of Cinchonidine’s action primarily involve the inhibition of the growth and multiplication of Plasmodium parasites within red blood cells . This inhibition results in a decrease in the severity of malaria symptoms and can potentially save lives . Additionally, Cinchonidine has been reported to exhibit cell-protective effects against certain types of cell damage .
Action Environment
The action, efficacy, and stability of Cinchonidine can be influenced by various environmental factors. For instance, the natural environmental conditions such as temperature and humidity, as well as the type of soil, can have a substantial impact on the germination of Cinchona plants, which produce Cinchonidine . This suggests that the production and availability of Cinchonidine can be influenced by environmental conditions, which in turn can affect its action and efficacy .
生化学分析
Biochemical Properties
Cinchonidine, like other Cinchona alkaloids, plays a significant role in biochemical reactions. It interacts with various enzymes, proteins, and other biomolecules . For instance, it has been found to exhibit cell-protective effects in indoxyl sulfate-stimulated human umbilical vein endothelial cells (HUVECs) .
Cellular Effects
Cinchonidine has been shown to have substantial effects on various types of cells and cellular processes. In HUVECs, it has been found to reverse indoxyl sulfate-induced cell death, cellular senescence, and impairment of tube formation .
Molecular Mechanism
Cinchonidine exerts its effects at the molecular level through various mechanisms. For instance, it has been found to downregulate the p53 signaling pathway in IS-treated HUVECs, promoting the degradation of p53 and the cytoplasmic-nuclear shuttling of MDM2 .
準備方法
Synthetic Routes and Reaction Conditions: Cinchonidine can be extracted from the bark of cinchona trees. The extraction process involves the use of solvents such as ethanol or methanol to isolate the alkaloid. The crude extract is then purified through crystallization or chromatography techniques.
Industrial Production Methods: Industrial production of cinchonidine involves the cultivation of cinchona trees, followed by the extraction and purification of the alkaloid. The process is optimized to ensure high yield and purity of the final product. Advanced techniques such as supercritical fluid extraction and high-performance liquid chromatography are employed to enhance efficiency .
化学反応の分析
Types of Reactions: Cinchonidine undergoes various chemical reactions, including:
Oxidation: Cinchonidine can be oxidized to form cinchonidine N-oxide.
Reduction: Reduction of cinchonidine can yield dihydrocinchonidine.
Substitution: Cinchonidine can undergo substitution reactions, particularly at the quinoline ring.
Common Reagents and Conditions:
Oxidation: Reagents such as hydrogen peroxide or m-chloroperbenzoic acid are used under mild conditions.
Reduction: Catalytic hydrogenation using palladium on carbon as a catalyst.
Substitution: Electrophilic reagents such as alkyl halides or acyl chlorides in the presence of a base.
Major Products:
Oxidation: Cinchonidine N-oxide.
Reduction: Dihydrocinchonidine.
Substitution: Various substituted quinoline derivatives.
類似化合物との比較
Cinchonidine is often compared with other cinchona alkaloids such as quinine, quinidine, and cinchonine. These compounds share similar structures but differ in their stereochemistry and pharmacological properties:
Quinine: Primarily used as an antimalarial agent.
Quinidine: Used as an antiarrhythmic agent.
Cinchonine: Similar to cinchonidine but with different stereochemistry.
Cinchonidine is unique in its ability to direct chirality in asymmetric synthesis and its broader range of applications in scientific research .
特性
CAS番号 |
485-71-2 |
---|---|
分子式 |
C19H22N2O |
分子量 |
294.4 g/mol |
IUPAC名 |
(R)-[(4S,5R)-5-ethenyl-1-azabicyclo[2.2.2]octan-2-yl]-quinolin-4-ylmethanol |
InChI |
InChI=1S/C19H22N2O/c1-2-13-12-21-10-8-14(13)11-18(21)19(22)16-7-9-20-17-6-4-3-5-15(16)17/h2-7,9,13-14,18-19,22H,1,8,10-12H2/t13-,14-,18?,19+/m0/s1 |
InChIキー |
KMPWYEUPVWOPIM-CZHSWUJBSA-N |
SMILES |
C=CC1CN2CCC1CC2C(C3=CC=NC4=CC=CC=C34)O |
異性体SMILES |
C=C[C@H]1CN2CC[C@H]1CC2[C@@H](C3=CC=NC4=CC=CC=C34)O |
正規SMILES |
C=CC1CN2CCC1CC2C(C3=CC=NC4=CC=CC=C34)O |
melting_point |
210.5 °C |
Key on ui other cas no. |
118-10-5 485-71-2 1042167-24-7 |
物理的記述 |
Solid |
ピクトグラム |
Irritant; Health Hazard |
溶解性 |
0.2 mg/mL at 25 °C |
同義語 |
2-Quinuclidinemethanol, alpha-4-quinolyl-5-vinyl- cinchonidine cinchonidine hydrochloride cinchonidine monohydrochloride cinchonidine sulfate(2:1) cinchonidine, (1beta,3alpha,4beta,8alpha,9R)-isome |
製品の起源 |
United States |
Retrosynthesis Analysis
AI-Powered Synthesis Planning: Our tool employs the Template_relevance Pistachio, Template_relevance Bkms_metabolic, Template_relevance Pistachio_ringbreaker, Template_relevance Reaxys, Template_relevance Reaxys_biocatalysis model, leveraging a vast database of chemical reactions to predict feasible synthetic routes.
One-Step Synthesis Focus: Specifically designed for one-step synthesis, it provides concise and direct routes for your target compounds, streamlining the synthesis process.
Accurate Predictions: Utilizing the extensive PISTACHIO, BKMS_METABOLIC, PISTACHIO_RINGBREAKER, REAXYS, REAXYS_BIOCATALYSIS database, our tool offers high-accuracy predictions, reflecting the latest in chemical research and data.
Strategy Settings
Precursor scoring | Relevance Heuristic |
---|---|
Min. plausibility | 0.01 |
Model | Template_relevance |
Template Set | Pistachio/Bkms_metabolic/Pistachio_ringbreaker/Reaxys/Reaxys_biocatalysis |
Top-N result to add to graph | 6 |
Feasible Synthetic Routes
Q1: What is the role of cinchonidine in heterogeneous catalysis?
A: Cinchonidine is a chiral modifier frequently employed in heterogeneous catalysis, especially in the enantioselective hydrogenation of prochiral ketones and α,β-unsaturated carboxylic acids. [, , , , , ] When adsorbed on metal surfaces like platinum or palladium, it creates a chiral environment that favors the formation of one enantiomer over the other. [, , ]
Q2: How does cinchonidine adsorb on metal surfaces like platinum?
A: Cinchonidine adsorbs on platinum surfaces primarily via its quinoline moiety. [, , ] The adsorption mode is coverage-dependent, with multiple species coexisting on the surface. [, , ] At low concentrations, a predominantly flat adsorption mode is observed, whereas at higher concentrations, tilted species, including α-H abstracted and N lone pair bonded cinchonidine, emerge. [, , ]
Q3: What is the significance of the different adsorption modes of cinchonidine?
A: The different adsorption modes of cinchonidine on platinum surfaces have significant implications for enantioselectivity. [] It is suggested that the flat-lying orientation of the quinoline ring, prevalent at intermediate concentrations, is associated with optimal activity and enantioselectivity in catalytic processes. []
Q4: How does the choice of solvent impact cinchonidine's behavior in heterogeneous catalysis?
A: The solvent plays a crucial role in cinchonidine adsorption and catalytic performance. [, ] Non-polar solvents, such as cyclohexane, allow adsorbed cinchonidine to remain on the platinum surface, while more polar solvents, like dichloromethane, facilitate its removal. [] This behavior correlates with cinchonidine solubility and the overall catalytic performance. [] For example, in the hydrogenation of ethyl pyruvate, ethanol as a solvent leads to higher enantioselectivity in a fixed-bed reactor compared to acetic acid, while the opposite is observed in a batch reactor. []
Q5: What is the role of hydrogen in cinchonidine adsorption and catalytic activity?
A: Hydrogen plays a critical role in preparing effective cinchonidine-modified platinum catalysts. [, ] Initially, it conditions the surface by reducing and removing contaminants, facilitating cinchonidine adsorption. [, ] Subsequently, hydrogen can hydrogenate the quinoline fragment of cinchonidine, promoting its desorption. [] Catalysts prepared and used under strictly anaerobic conditions display negligible activity and enantioselectivity, highlighting the importance of oxygen in successful catalyst preparation. []
Q6: How does the size of platinum particles affect cinchonidine's adsorption and catalytic activity?
A: The size of platinum particles influences the distribution of different cinchonidine conformations on the surface. [, ] Smaller particles, or those where platinum domains are reduced by alloying with gold, tend to disfavor the formation of flat-lying cinchonidine species. [, ] This can impact the enantioselectivity of the catalyst.
Q7: Can cinchonidine be immobilized on a support for heterogeneous catalysis?
A: Yes, cinchonidine can be immobilized on supports like silica for heterogeneous catalysis. [, ] One strategy involves functionalizing cinchonidine with a triethoxysilane group for subsequent attachment to silica. [] This immobilization can lead to catalysts with comparable or even superior performance to systems where cinchonidine is added directly to the reaction mixture. []
Q8: Are there other molecules besides cinchonidine used as chiral modifiers in heterogeneous catalysis?
A: Yes, various molecules besides cinchonidine are used as chiral modifiers, including O-phenyl derivatives of cinchonidine, (R)-2-(pyrrolidin-1-yl)-1-(1-naphthyl)ethanol (PNE), and synthetic ligands like quiphos. [, , ] The structure and properties of the modifier can significantly impact the adsorption mode and the resulting enantioselectivity of the catalyst. [, ]
Q9: What is the molecular formula and weight of cinchonidine?
A9: The molecular formula of cinchonidine is C19H22N2O, and its molecular weight is 294.38 g/mol.
Q10: What spectroscopic techniques are typically used to characterize cinchonidine and its interactions?
A10: Several spectroscopic techniques are employed to characterize cinchonidine, including:
- Nuclear Magnetic Resonance (NMR): This technique is essential for determining the structure, conformation, and interactions of cinchonidine in solution. [, , , , ] It provides information about the spatial arrangement of atoms, hydrogen bonding, and the presence of different conformers. [, , , , ]
- Infrared Spectroscopy (IR): IR spectroscopy, including attenuated total reflection infrared (ATR-IR) spectroscopy, is used to study the adsorption of cinchonidine on metal surfaces. [, , , , , ] It provides insights into the binding modes of cinchonidine and its interactions with the surface and other molecules. [, , , , , ]
- Surface-Enhanced Raman Spectroscopy (SERS): This technique provides enhanced sensitivity for studying adsorbed species on metal surfaces, enabling the investigation of cinchonidine's orientation and interactions on platinum. [, ]
Q11: How does modification of the cinchonidine structure affect its activity and selectivity?
A: Modifying the cinchonidine structure can significantly impact its performance as a chiral modifier. [, , , , , ] For example:
- C9-O-substitution: Introducing bulky substituents at the C9-OH group can lead to lower enantioselectivity and even reverse the enantiomeric preference in some cases. []
- Quinuclidine nitrogen modification: Altering the quinuclidine nitrogen, such as through quaternization, can impact its interaction with the substrate and the metal surface, influencing both activity and selectivity. [, , ]
- Hydrogenation of the quinoline ring: Hydrogenating the quinoline ring can affect its adsorption geometry and interaction with the metal surface, impacting the enantioselectivity. [, ]
Q12: What is the role of computational chemistry in understanding cinchonidine's behavior?
A: Computational chemistry, particularly Density Functional Theory (DFT) calculations, has proven valuable in understanding cinchonidine's behavior. [, , , , ] These calculations provide insights into:
- Conformational analysis: Identifying and analyzing the stability of different cinchonidine conformations in solution and on surfaces. [, , , ]
- Adsorption energies: Determining the strength of interaction between cinchonidine and metal surfaces in various orientations. [, ]
- Mechanistic understanding: Proposing and evaluating models for the interaction of cinchonidine with substrates like pyruvate and the origin of enantioselectivity in catalytic reactions. []
Q13: What are some other applications of cinchonidine beyond heterogeneous catalysis?
A13: Apart from its role in catalysis, cinchonidine finds applications in:
- Chiral resolution: It can be used as a resolving agent to separate enantiomers of chiral compounds through the formation of diastereomeric salts. []
Q14: What analytical techniques are used to quantify cinchonidine?
A: High-performance liquid chromatography (HPLC) is commonly employed for the quantification of cinchonidine in various matrices. [, , ] This technique allows for separation and detection of cinchonidine, even in complex mixtures.
Q15: What are some of the challenges and future directions in cinchonidine research?
A15: Despite extensive research, several challenges and exciting research directions remain in the field of cinchonidine, including:
- Elucidating the precise mechanism of enantioselection: While various models have been proposed, the exact mechanism by which cinchonidine induces enantioselectivity in heterogeneous catalysis is still debated and requires further investigation. [, ]
- Designing more efficient and selective catalysts: Developing new synthetic methodologies and strategies to immobilize cinchonidine on various supports with improved control over its orientation and accessibility could lead to more efficient and selective catalysts. []
- Exploring alternative applications: Given its diverse properties, investigating other potential applications of cinchonidine, particularly in the biomedical field, holds promise. [, ] This requires a deeper understanding of its biological activity, toxicity, and pharmacokinetic properties.
試験管内研究製品の免責事項と情報
BenchChemで提示されるすべての記事および製品情報は、情報提供を目的としています。BenchChemで購入可能な製品は、生体外研究のために特別に設計されています。生体外研究は、ラテン語の "in glass" に由来し、生物体の外で行われる実験を指します。これらの製品は医薬品または薬として分類されておらず、FDAから任何の医療状態、病気、または疾患の予防、治療、または治癒のために承認されていません。これらの製品を人間または動物に体内に導入する形態は、法律により厳格に禁止されています。これらのガイドラインに従うことは、研究と実験において法的および倫理的な基準の遵守を確実にするために重要です。