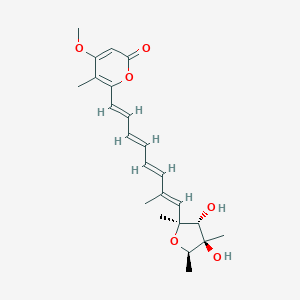
シトレオビリジン
概要
説明
科学的研究の応用
Toxicokinetics of Citreoviridin
Citreoviridin is known for its high bioavailability and persistence in biological systems. A study demonstrated that citreoviridin exhibited a bioavailability of approximately 116.4% in swine, indicating significant absorption and retention in the body . The compound's elimination half-life was found to be around 21.4 hours, with a large volume of distribution (1.7 L), suggesting that it can accumulate in tissues such as the liver, kidney, and heart .
The permeability of citreoviridin across intestinal cells was assessed using Caco-2 cell lines, revealing a high permeability coefficient that correlates with its potential bioavailability in humans . This suggests that citreoviridin may pose health risks due to its accumulation and prolonged presence in the body.
Biological Effects and Mechanisms
Citreoviridin has been studied for its effects on various cellular processes, particularly concerning vascular health and bone metabolism:
- Inhibition of Calcium Deposition : Recent research indicated that low concentrations of citreoviridin can prevent intracellular calcium deposition in vascular smooth muscle cells (vSMCs) induced by angiotensin II (Ang II). This effect is crucial as excessive calcium deposition is linked to vascular calcification .
- Osteoclast Differentiation : Citreoviridin also inhibits osteoclast differentiation, which is essential for bone remodeling. It was shown to reduce the activity of osteoclasts stimulated by receptor activator of nuclear factor kappa-Β ligand (RANKL), indicating a potential application in treating osteoporosis .
- Oxidative Stress Induction : Citreoviridin induces apoptosis through oxidative damage mechanisms. Studies have shown that it can increase reactive oxygen species (ROS) production, leading to cellular stress and apoptosis in various cell types .
Case Studies
Several studies have documented the effects of citreoviridin on different cell types:
- Vascular Smooth Muscle Cells :
- Osteoclast Activity :
- Toxicokinetics Analysis :
作用機序
シトレオビリジンは、細胞のエネルギー産生に不可欠なミトコンドリアATPシンターゼ系を阻害することによってその効果を発揮します . この阻害は、ATPレベルの低下につながり、細胞機能不全と死を引き起こします。 この化合物は、ヒト肝細胞におけるリソソーム-ミトコンドリア軸を介してオートファジー依存性アポトーシスも誘導します . 分子標的は、ミトコンドリアATPシンターゼとオートファジーおよびアポトーシス経路に関与するさまざまなタンパク質が含まれます .
生化学分析
Biochemical Properties
Citreoviridin acts as a potent inhibitor of the soluble mitochondrial ATPase (adenosine triphosphatase), similar to the closely related aurovertins B and D . It inhibits several mitochondrial energy-linked reactions, including ADP-stimulated respiration in whole mitochondria from ox heart and rat liver, ATP-driven reduction of NAD+ by succinate, ATP-driven NAD transhydrogenase, and ATPase from ox heart submitochondrial particles .
Cellular Effects
Citreoviridin has been shown to induce autophagy-dependent apoptosis through the lysosomal-mitochondrial axis in human liver HepG2 cells . It also significantly augments atherosclerotic plaques and increases expressions of ICAM-1, VCAM-1, VEGF, and ET-1 in aorta . The activation of NF-κB was significantly elevated by citreoviridin treatment, indicating its effect on atherosclerosis may be regulated by activation of NF-κB .
Molecular Mechanism
Citreoviridin’s molecular mechanism of action involves the inhibition of the mitochondrial ATPase, leading to disruption of energy-linked reactions . It also induces apoptosis through the lysosomal-mitochondrial axis in HepG2 cells . Furthermore, it upregulates inflammation and endothelial dysfunction via the activation of NF-κB .
Temporal Effects in Laboratory Settings
It has been suggested that citreoviridin is easily absorbed in swine and persists in the body for a long duration due to its slow metabolism .
Dosage Effects in Animal Models
It has been reported that citreoviridin has a high bioavailability in swine .
Metabolic Pathways
The metabolic pathways of citreoviridin involve hydroxylation, methylation, desaturation, and dihydroxylation . The expression of these four homologous genes were highly correlated with citreoviridin production, suggesting that they may play an important role in citreoviridin biosynthesis .
Transport and Distribution
Citreoviridin has a large volume of distribution and a long elimination half-life in intravenous administration, suggesting the possibility of a slow metabolism of citreoviridin .
Subcellular Localization
Given its role as an inhibitor of mitochondrial ATPase, it is likely that citreoviridin localizes to the mitochondria where it exerts its effects .
準備方法
合成経路と反応条件: シトレオビリジンは、高度に還元されたポリケチド産物です。 その合成には、アセチルおよびマロニルサブユニットの重合によってポリケチドの形成を触媒する酵素であるポリケチドシンターゼの使用が含まれます . 生合成経路には、水酸化、メチル化、脱飽和、ジヒドロキシル化などのいくつかのステップが含まれます .
工業生産方法: シトレオビリジンの工業生産には、通常、マイコトキシン収量を最大化するために、制御された条件下でペニシリウム・シトレオニグラムを培養することが含まれます。 菌類は栄養豊富な培地で生育され、シトレオビリジンはさまざまなクロマトグラフィー技術を使用して抽出および精製されます .
化学反応の分析
反応の種類: シトレオビリジンは、酸化、還元、置換など、いくつかの種類の化学反応を受けます。これらの反応は、さまざまな試薬と条件によって促進されます。
一般的な試薬と条件:
酸化: シトレオビリジンは、過酸化水素または過マンガン酸カリウムなどの試薬を使用して酸化できます。
還元: 還元反応は、水素化ホウ素ナトリウムまたは水素化アルミニウムリチウムなどの還元剤を使用して実行できます。
置換: 置換反応には、アミンまたはチオールなどの求核剤が関与することがよくあります。
主要な製品: これらの反応から生成される主要な生成物には、シトレオビリジンのヒドロキシル化、メチル化、脱飽和誘導体が含まれます .
4. 科学研究アプリケーション
シトレオビリジンは、さまざまな分野にわたるいくつかの科学研究アプリケーションを持っています。
化学:
- ポリケチドの生合成を研究するためのモデル化合物として使用されます。
- ポリケチド合成の合成方法の開発に使用されています .
生物学:
医学:
- 菌類汚染による病気の治療法を開発するための潜在的な用途を探っています。
- マイコトキシン誘発性疾患のメカニズムを理解するための研究で使用されています .
産業:
- 食品中のマイコトキシン検出方法の開発に適用されます。
- 農産物の菌類汚染の研究に使用されています .
類似化合物との比較
シトレオビリジンは、α-ピロンポリエン構造を共有するF1-ATPaseβサブユニット阻害剤のクラスに属します。同様の化合物には以下が含まれます。
アウロベルチン: ミトコンドリアATPシンターゼを阻害し、同様の毒性効果を持つ別のポリケチド.
ベルコシジン: 構造と作用機序が類似したマイコトキシン.
アステルトキシン: 構造的類似性を共有し、ミトコンドリア機能を阻害します.
ユニークさ: シトレオビリジンは、ペニシリウム・シトレオニグラムによる特定の産生と、ミトコンドリアATPシンターゼに対する強力な阻害効果のためにユニークです。 オートファジー依存性アポトーシスを誘導する能力も、それを他の類似化合物から際立たせています .
生物活性
Citreoviridin (CIT), a mycotoxin primarily produced by the fungus Penicillium citreonigrum, has garnered attention due to its significant biological activity and health implications. This article delves into the biological properties, toxicokinetics, binding interactions, and associated health risks of citreoviridin, supported by recent research findings and data.
Overview of Citreoviridin
Citreoviridin is known as a potent mycotoxin found in various agricultural products, especially rice. It has been linked to severe health issues, including acute cardiac beriberi, characterized by neurological symptoms and cardiovascular complications. The compound's toxicological profile necessitates a comprehensive understanding of its biological activity.
Toxicokinetics
Recent studies have elucidated the toxicokinetics of citreoviridin through both in vivo and in vitro experiments.
Key Findings:
- Bioavailability : Citreoviridin exhibits high bioavailability in swine, with a reported absorption rate of 116.4% when administered intravenously or orally .
- Metabolism : The metabolic pathways of citreoviridin include hydroxylation, methylation, desaturation, and dihydroxylation. Notably, human liver S9 fractions produce higher levels of certain metabolites compared to swine .
- Elimination Half-Life : The compound has a relatively long elimination half-life in swine, suggesting it is poorly metabolized in the liver, with traces detectable even after 52 hours post-administration .
Table 1: Summary of Toxicokinetic Parameters
Parameter | Value |
---|---|
Bioavailability (Swine) | 116.4% |
Elimination Half-Life (Swine) | >40 hours |
Metabolite Production | Hydroxylation, Methylation, etc. |
Binding Interactions
Citreoviridin's interaction with human serum albumin (HSA) has been extensively studied to understand its transport and bioavailability in the human body.
Key Observations:
- Binding Mechanism : Citreoviridin binds to HSA through hydrophobic interactions and hydrogen bonding. The binding site is located within the hydrophobic pocket of HSA .
- Structural Changes : The presence of citreoviridin alters the secondary structure of HSA, as evidenced by changes observed in UV-Vis and circular dichroism spectra .
Health Implications
Citreoviridin poses significant health risks due to its toxic effects. It has been associated with acute cardiac beriberi outbreaks in rice-producing regions.
Case Studies:
- Acute Cardiac Beriberi : Historical reports indicate that citreoviridin exposure led to outbreaks characterized by severe neurological symptoms such as convulsions and respiratory arrest .
- Animal Studies : Experimental studies on rats and guinea pigs have shown that citreoviridin induces toxic effects similar to those observed in humans during beriberi outbreaks .
特性
IUPAC Name |
6-[(1E,3E,5E,7E)-8-[(2S,3R,4R,5R)-3,4-dihydroxy-2,4,5-trimethyloxolan-2-yl]-7-methylocta-1,3,5,7-tetraenyl]-4-methoxy-5-methylpyran-2-one | |
---|---|---|
Source | PubChem | |
URL | https://pubchem.ncbi.nlm.nih.gov | |
Description | Data deposited in or computed by PubChem | |
InChI |
InChI=1S/C23H30O6/c1-15(14-22(4)21(25)23(5,26)17(3)29-22)11-9-7-8-10-12-18-16(2)19(27-6)13-20(24)28-18/h7-14,17,21,25-26H,1-6H3/b8-7+,11-9+,12-10+,15-14+/t17-,21+,22+,23+/m1/s1 | |
Source | PubChem | |
URL | https://pubchem.ncbi.nlm.nih.gov | |
Description | Data deposited in or computed by PubChem | |
InChI Key |
JLSVDPQAIKFBTO-OMCRQDLASA-N | |
Source | PubChem | |
URL | https://pubchem.ncbi.nlm.nih.gov | |
Description | Data deposited in or computed by PubChem | |
Canonical SMILES |
CC1C(C(C(O1)(C)C=C(C)C=CC=CC=CC2=C(C(=CC(=O)O2)OC)C)O)(C)O | |
Source | PubChem | |
URL | https://pubchem.ncbi.nlm.nih.gov | |
Description | Data deposited in or computed by PubChem | |
Isomeric SMILES |
C[C@@H]1[C@]([C@H]([C@](O1)(C)/C=C(\C)/C=C/C=C/C=C/C2=C(C(=CC(=O)O2)OC)C)O)(C)O | |
Source | PubChem | |
URL | https://pubchem.ncbi.nlm.nih.gov | |
Description | Data deposited in or computed by PubChem | |
Molecular Formula |
C23H30O6 | |
Source | PubChem | |
URL | https://pubchem.ncbi.nlm.nih.gov | |
Description | Data deposited in or computed by PubChem | |
DSSTOX Substance ID |
DTXSID301017584 | |
Record name | Citreoviridin | |
Source | EPA DSSTox | |
URL | https://comptox.epa.gov/dashboard/DTXSID301017584 | |
Description | DSSTox provides a high quality public chemistry resource for supporting improved predictive toxicology. | |
Molecular Weight |
402.5 g/mol | |
Source | PubChem | |
URL | https://pubchem.ncbi.nlm.nih.gov | |
Description | Data deposited in or computed by PubChem | |
CAS No. |
25425-12-1 | |
Record name | Citreoviridin | |
Source | CAS Common Chemistry | |
URL | https://commonchemistry.cas.org/detail?cas_rn=25425-12-1 | |
Description | CAS Common Chemistry is an open community resource for accessing chemical information. Nearly 500,000 chemical substances from CAS REGISTRY cover areas of community interest, including common and frequently regulated chemicals, and those relevant to high school and undergraduate chemistry classes. This chemical information, curated by our expert scientists, is provided in alignment with our mission as a division of the American Chemical Society. | |
Explanation | The data from CAS Common Chemistry is provided under a CC-BY-NC 4.0 license, unless otherwise stated. | |
Record name | Citreoviridin | |
Source | ChemIDplus | |
URL | https://pubchem.ncbi.nlm.nih.gov/substance/?source=chemidplus&sourceid=0025425121 | |
Description | ChemIDplus is a free, web search system that provides access to the structure and nomenclature authority files used for the identification of chemical substances cited in National Library of Medicine (NLM) databases, including the TOXNET system. | |
Record name | Citreoviridin | |
Source | EPA DSSTox | |
URL | https://comptox.epa.gov/dashboard/DTXSID301017584 | |
Description | DSSTox provides a high quality public chemistry resource for supporting improved predictive toxicology. | |
Record name | Citreoviridin | |
Source | European Chemicals Agency (ECHA) | |
URL | https://echa.europa.eu/information-on-chemicals | |
Description | The European Chemicals Agency (ECHA) is an agency of the European Union which is the driving force among regulatory authorities in implementing the EU's groundbreaking chemicals legislation for the benefit of human health and the environment as well as for innovation and competitiveness. | |
Explanation | Use of the information, documents and data from the ECHA website is subject to the terms and conditions of this Legal Notice, and subject to other binding limitations provided for under applicable law, the information, documents and data made available on the ECHA website may be reproduced, distributed and/or used, totally or in part, for non-commercial purposes provided that ECHA is acknowledged as the source: "Source: European Chemicals Agency, http://echa.europa.eu/". Such acknowledgement must be included in each copy of the material. ECHA permits and encourages organisations and individuals to create links to the ECHA website under the following cumulative conditions: Links can only be made to webpages that provide a link to the Legal Notice page. | |
Record name | CITREOVIRIDIN | |
Source | FDA Global Substance Registration System (GSRS) | |
URL | https://gsrs.ncats.nih.gov/ginas/app/beta/substances/OWX7Q6CF4F | |
Description | The FDA Global Substance Registration System (GSRS) enables the efficient and accurate exchange of information on what substances are in regulated products. Instead of relying on names, which vary across regulatory domains, countries, and regions, the GSRS knowledge base makes it possible for substances to be defined by standardized, scientific descriptions. | |
Explanation | Unless otherwise noted, the contents of the FDA website (www.fda.gov), both text and graphics, are not copyrighted. They are in the public domain and may be republished, reprinted and otherwise used freely by anyone without the need to obtain permission from FDA. Credit to the U.S. Food and Drug Administration as the source is appreciated but not required. | |
Retrosynthesis Analysis
AI-Powered Synthesis Planning: Our tool employs the Template_relevance Pistachio, Template_relevance Bkms_metabolic, Template_relevance Pistachio_ringbreaker, Template_relevance Reaxys, Template_relevance Reaxys_biocatalysis model, leveraging a vast database of chemical reactions to predict feasible synthetic routes.
One-Step Synthesis Focus: Specifically designed for one-step synthesis, it provides concise and direct routes for your target compounds, streamlining the synthesis process.
Accurate Predictions: Utilizing the extensive PISTACHIO, BKMS_METABOLIC, PISTACHIO_RINGBREAKER, REAXYS, REAXYS_BIOCATALYSIS database, our tool offers high-accuracy predictions, reflecting the latest in chemical research and data.
Strategy Settings
Precursor scoring | Relevance Heuristic |
---|---|
Min. plausibility | 0.01 |
Model | Template_relevance |
Template Set | Pistachio/Bkms_metabolic/Pistachio_ringbreaker/Reaxys/Reaxys_biocatalysis |
Top-N result to add to graph | 6 |
Feasible Synthetic Routes
A: Citreoviridin is a potent inhibitor of mitochondrial F1F0-ATP synthase, specifically targeting the β-subunit. [] This interaction disrupts ATP synthesis, a critical process for cellular energy production. [, ] Downstream effects include:
- Inhibition of mitochondrial energy-linked reactions: This includes ADP-stimulated respiration, ATP-driven reduction of NAD+, and ATP-driven NAD transhydrogenase. []
- Induction of the unfolded protein response (UPR): This cellular stress response is triggered by the accumulation of unfolded proteins, leading to cell cycle arrest and inhibition of proliferation. []
- Increased reactive oxygen species (ROS) production: This contributes to oxidative stress, further enhancing UPR and leading to cell death. []
- Lysosomal membrane permeabilization: This releases cathepsin D, a protease that contributes to apoptosis. []
- Mitochondrial transmembrane potential collapse: This is a hallmark of mitochondrial dysfunction and contributes to apoptosis. []
ANone: Citreoviridin's structural characteristics are:
- Molecular Formula: C23H30O6 [, ]
- Molecular Weight: 402.48 g/mol [, ]
- Spectroscopic data: Citreoviridin exhibits characteristic UV/Vis, FT-IR, 1H-NMR, 13C-NMR, LC-MS/MS, and LC-MSD TOF spectral data. [, , ]
A: Citreoviridin itself doesn't possess catalytic properties. Its primary mode of action is the inhibition of F1F0-ATP synthase. [, ]
A: While computational studies specifically on Citreoviridin are limited in the provided research, mathematical modeling has been used to construct response networks illustrating the relationship between phosphorylated heat shock protein 90 β and the mitogen-activated protein kinase signaling pathway following Citreoviridin treatment. []
A: Modifications to Citreoviridin's structure, such as acetylation, decrease its inhibitory potency against F1F0-ATPase. [] Monoacetylation has a less pronounced effect compared to diacetylation, highlighting the importance of specific functional groups for activity. [] Hydrogenation of Citreoviridin monoacetate also diminishes its inhibitory potency. []
A: Citreoviridin shows good stability when stored frozen and protected from light for up to 10 months. [] Isomerization can occur with prolonged storage. [] Specific formulation strategies to enhance stability, solubility, or bioavailability are not discussed in the provided research.
A: Studies in swine revealed high bioavailability of Citreoviridin (116.4%) and a long elimination half-life (17.7 ± 3.3 h), suggesting slow metabolism. [] In vitro studies using Caco-2 cells indicated high intestinal permeability, suggesting easy absorption in the human intestine. [, ]
ANone: The provided research doesn't discuss resistance mechanisms to Citreoviridin or its potential cross-resistance with other compounds.
A: Citreoviridin is a mycotoxin with established toxicity. * Animal studies: In mice, near-lethal doses cause decreased motor activity, hypothermia, and catalepsy. [] Intravenous administration in rabbits leads to respiratory and cardiovascular failure. []* Cellular effects: Citreoviridin induces mitochondrial damage, leading to swelling, cristae breakage, and vacuolation. [] It also causes DNA damage in a dose-dependent manner. [] * Human health concerns: Citreoviridin is linked to acute cardiac beriberi, a potentially fatal condition. [, ]
ANone: The provided research does not discuss specific drug delivery or targeting strategies for Citreoviridin.
A: While specific biomarkers for Citreoviridin's effects are not identified in the provided research, DNA damage assessed by the comet assay (single-cell gel electrophoresis) has been explored as a potential biomarker for epidemiological studies. []
A: Various analytical techniques are employed for Citreoviridin analysis:* Chromatographic techniques: High-performance liquid chromatography (HPLC) coupled with various detectors like UV/Vis (PDA), fluorescence, and mass spectrometry (MS/MS, TOF) are widely used. [, , , , , , ] * Spectroscopic techniques: UV/Vis, FT-IR, 1H-NMR, and 13C-NMR are utilized for structural characterization. [, , ]* Thin-layer chromatography (TLC): This technique is used for rapid detection and quantification. []
ANone: The provided research doesn't provide information about the environmental impact or degradation of Citreoviridin.
ANone: The provided research primarily focuses on Citreoviridin's extraction, purification, and analysis, without specific details on its dissolution or solubility in various media.
A: Analytical methods for Citreoviridin detection and quantification, particularly HPLC-based methods, are validated for parameters like linearity, sensitivity (limit of detection), recovery, and precision (repeatability). [, , , , ]
ANone: The provided research focuses on the analytical aspects and biological effects of Citreoviridin without delving into quality control and assurance measures during development, manufacturing, or distribution.
A: Citreoviridin research has a significant history:* 1947: First isolated in Japan during investigations of "yellow rice" disease, linked to cardiac beriberi. [, ]* 1970s - 1980s: Studies focused on elucidating its structure, biosynthetic pathway, and mechanism of action as an F1F0-ATPase inhibitor. [, , ]* 2006: Implicated in a beriberi outbreak in Brazil, renewing interest in its toxicology and occurrence in food. []* Recent years: Research focuses on understanding its molecular mechanisms of toxicity, potential as an anti-cancer agent, and developing sensitive analytical methods for detection. [, , , , , ]
ANone: Citreoviridin research exemplifies cross-disciplinary collaboration:
- Mycology and Food Science: Studying Citreoviridin-producing fungi, contamination routes in crops, and developing strategies for prevention. [, , , ]
- Analytical Chemistry: Developing and validating sensitive, specific, and robust methods for Citreoviridin detection and quantification in various matrices. [, , , , ]
- Toxicology and Pharmacology: Investigating Citreoviridin's mechanisms of toxicity, target organs, and potential long-term health effects. [, , , ]
- Biochemistry and Molecular Biology: Elucidating Citreoviridin's interaction with F1F0-ATPase, downstream signaling pathways, and cellular responses. [, , , , ]
- Medicinal Chemistry: Exploring Citreoviridin's potential as a lead compound for developing novel therapeutics, particularly in cancer and diseases involving ectopic ATP synthase. [, ]
試験管内研究製品の免責事項と情報
BenchChemで提示されるすべての記事および製品情報は、情報提供を目的としています。BenchChemで購入可能な製品は、生体外研究のために特別に設計されています。生体外研究は、ラテン語の "in glass" に由来し、生物体の外で行われる実験を指します。これらの製品は医薬品または薬として分類されておらず、FDAから任何の医療状態、病気、または疾患の予防、治療、または治癒のために承認されていません。これらの製品を人間または動物に体内に導入する形態は、法律により厳格に禁止されています。これらのガイドラインに従うことは、研究と実験において法的および倫理的な基準の遵守を確実にするために重要です。