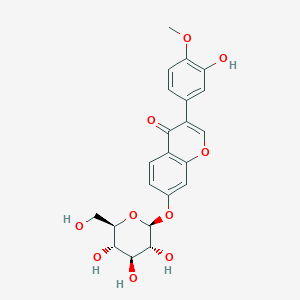
カリコシン7-O-グルコシド
概要
説明
カリコシン-7-O-β-D-グルコシドは、伝統的な薬草であるオウギ(Astragalus membranaceus)の根から単離されたイソフラボン配糖体です。 この化合物は、神経保護、心臓保護、抗炎症、抗酸化作用など、さまざまな生物活性で知られています 。 分子式はC22H22O10、分子量は446.40 g/molです .
2. 製法
合成経路と反応条件: カリコシン-7-O-β-D-グルコシドは、グルコシルトランスフェラーゼ酵素を含む生触媒法によって合成できます。 そのような方法の1つは、ダイズ(Glycine max)から単離されたウリジン二リン酸依存性グルコシルトランスフェラーゼ(UGT)を使用し、カリコシンのC7ヒドロキシル基を選択的にグルコシル化するものです 。 この反応は、UGTをスクロースシンターゼと結合させて、スクロースからウリジン二リン酸グルコースを再利用することで最適化されます .
工業生産方法: カリコシン-7-O-β-D-グルコシドの工業生産は、通常、オウギの根からの抽出によるものです。抽出プロセスには、根を乾燥させ、メタノールまたはエタノールを使用して溶媒抽出を行うことが含まれます。 次に、抽出物をクロマトグラフィー技術を使用して精製し、目的の化合物を単離します .
科学的研究の応用
Calycosin-7-O-beta-D-glucoside has a wide range of scientific research applications:
作用機序
カリコシン-7-O-β-D-グルコシドは、さまざまな分子標的と経路を通じて効果を発揮します。
神経保護: マトリックスメタロプロテイナーゼの発現と活性を阻害し、微小血管におけるカベオリン-1とタイト結合タンパク質の発現を維持します.
心臓保護: この化合物は、虚血再灌流モデルにおいて、梗塞容積、組織学的損傷、血液脳関門の透過性を軽減します.
抗炎症: インターロイキン-10/JAK2/STAT3シグナル伝達経路を調節し、心筋を虚血再灌流誘発損傷から保護します.
6. 類似の化合物との比較
カリコシン-7-O-β-D-グルコシドは、その特定の生物活性と分子構造により、イソフラボン配糖体の中でユニークな存在です。類似の化合物には以下のようなものがあります。
オノニン: 同様の抗酸化作用と抗炎症作用を持つ別のイソフラボン配糖体です.
フォルモノネチン: 神経保護および心臓保護作用を持つイソフラボンです.
ビオチャニンA: 抗がん作用と抗炎症作用で知られています.
比較すると、カリコシン-7-O-β-D-グルコシドは、神経保護および心臓保護におけるより高い効力と、より幅広い生物活性によって際立っています .
生化学分析
Biochemical Properties
Calycosin 7-O-glucoside interacts with various enzymes and proteins in biochemical reactions. For instance, it is synthesized by the enzyme uridine diphosphate-dependent glucosyltransferase (UGT) UGT88E18, which selectively and effectively glucosylates the C7 hydroxyl group of calycosin .
Cellular Effects
Calycosin 7-O-glucoside has significant effects on various types of cells and cellular processes. It influences cell function, including impact on cell signaling pathways, gene expression, and cellular metabolism .
Molecular Mechanism
At the molecular level, Calycosin 7-O-glucoside exerts its effects through binding interactions with biomolecules, enzyme inhibition or activation, and changes in gene expression .
Dosage Effects in Animal Models
The effects of Calycosin 7-O-glucoside vary with different dosages in animal models. Studies are ongoing to determine any threshold effects observed in these studies, as well as any toxic or adverse effects at high doses .
Transport and Distribution
Calycosin 7-O-glucoside is transported and distributed within cells and tissues. The specific transporters or binding proteins that it interacts with, as well as any effects on its localization or accumulation, are currently being studied .
Subcellular Localization
This includes any targeting signals or post-translational modifications that direct it to specific compartments or organelles .
準備方法
Synthetic Routes and Reaction Conditions: Calycosin-7-O-beta-D-glucoside can be synthesized through biocatalytic methods involving glucosyltransferase enzymes. One such method involves the use of uridine diphosphate-dependent glucosyltransferase (UGT) isolated from Glycine max, which selectively glucosylates the C7 hydroxyl group of calycosin . The reaction is optimized by coupling UGT with sucrose synthase to recycle uridine diphosphate glucose from sucrose .
Industrial Production Methods: Industrial production of Calycosin-7-O-beta-D-glucoside typically involves extraction from Astragalus membranaceus roots. The extraction process includes drying the roots, followed by solvent extraction using methanol or ethanol. The extract is then purified using chromatographic techniques to isolate the desired compound .
化学反応の分析
反応の種類: カリコシン-7-O-β-D-グルコシドは、以下を含むさまざまな化学反応を起こします。
酸化: この反応は、キノンなどの酸化された誘導体の生成につながる可能性があります。
還元: 還元反応は、化合物を対応するジヒドロ誘導体に変換できます。
置換: 置換反応はヒドロキシル基で起こり、さまざまな誘導体の生成につながります。
一般的な試薬と条件:
酸化: 一般的な酸化剤には、過酸化水素と過マンガン酸カリウムがあります。
還元: 水素化ホウ素ナトリウムや水素化リチウムアルミニウムなどの還元剤が使用されます。
置換: 無水酢酸やベンゾイルクロリドなどの試薬は、それぞれアセチル化反応やベンゾイル化反応に使用されます。
主要な生成物:
酸化: キノンなどの酸化された誘導体。
還元: ジヒドロ誘導体。
置換: アセチル化およびベンゾイル化誘導体。
4. 科学研究への応用
カリコシン-7-O-β-D-グルコシドは、幅広い科学研究への応用があります。
類似化合物との比較
Calycosin-7-O-beta-D-glucoside is unique among isoflavonoid glucosides due to its specific biological activities and molecular structure. Similar compounds include:
Ononin: Another isoflavonoid glucoside with similar antioxidant and anti-inflammatory properties.
Formononetin: An isoflavonoid with neuroprotective and cardioprotective effects.
Biochanin A: Known for its anti-cancer and anti-inflammatory activities.
In comparison, Calycosin-7-O-beta-D-glucoside stands out due to its higher potency in neuroprotection and cardioprotection, as well as its broader range of biological activities .
生物活性
The compound 3-(3-hydroxy-4-methoxyphenyl)-7-[(2S,3R,4S,5S,6R)-3,4,5-trihydroxy-6-(hydroxymethyl)oxan-2-yl]oxychromen-4-one , also known as Neodiosmin (CAS No. 20633-67-4), is a flavone glycoside that has garnered attention for its diverse biological activities. This article reviews the compound's biological activity based on recent research findings.
Chemical Structure and Properties
Neodiosmin is characterized by its complex structure which includes a chromone backbone and multiple hydroxyl groups. Its molecular formula is , with a molecular weight of 592.55 g/mol . The presence of these functional groups contributes to its biological activities.
Anticancer Activity
Neodiosmin and its derivatives have shown significant anticancer properties . Research indicates that chromone derivatives exhibit cytotoxic effects against various cancer cell lines, including leukemia and breast cancer cells. For instance, one study reported IC50 values of 22.09 µg/mL and 6.40 µg/mL against specific cancer cell lines, indicating potent cytotoxicity . The mechanism involves the induction of apoptosis and inhibition of cell proliferation through multiple signaling pathways.
Neuroprotective Effects
Recent studies suggest that Neodiosmin enhances neuroplasticity and exhibits neuroprotective effects. It has been shown to promote the proliferation of hippocampal neurons and increase the phosphorylation of cAMP response element-binding protein (CREB), which is crucial for neuronal survival and synaptogenesis . These findings highlight its potential in treating neurodegenerative diseases.
Antioxidant Activity
Neodiosmin also demonstrates antioxidant properties , which are vital for protecting cells from oxidative stress. In vitro assays have shown that it effectively scavenges free radicals and enhances total antioxidant capacity . This activity is attributed to its ability to modulate various biochemical pathways involved in oxidative stress responses.
Antimicrobial Activity
The compound has been evaluated for its antimicrobial properties against several pathogens. Preliminary results indicate significant inhibitory effects on bacterial strains, suggesting its potential as a natural antimicrobial agent .
The biological activities of Neodiosmin can be attributed to several mechanisms:
- Apoptosis Induction : Activation of apoptotic pathways in cancer cells.
- Neuroprotective Signaling : Modulation of CREB phosphorylation and brain-derived neurotrophic factor (BDNF) levels.
- Antioxidative Mechanisms : Scavenging of reactive oxygen species (ROS) and enhancement of cellular antioxidant defenses.
- Inhibition of Pathogen Growth : Disruption of microbial cell walls or metabolic pathways.
Case Studies
- Cytotoxicity in Cancer Cells : A study synthesized various chromone derivatives and tested their cytotoxicity against human cancer cell lines, demonstrating that modifications in the chromone structure significantly affect their anticancer potency .
- Neuroprotection in Animal Models : In vivo studies have shown that Neodiosmin administration leads to improved cognitive functions in animal models subjected to neurotoxic insults, suggesting its therapeutic potential for neurodegenerative diseases .
Data Summary
特性
IUPAC Name |
3-(3-hydroxy-4-methoxyphenyl)-7-[(2S,3R,4S,5S,6R)-3,4,5-trihydroxy-6-(hydroxymethyl)oxan-2-yl]oxychromen-4-one | |
---|---|---|
Source | PubChem | |
URL | https://pubchem.ncbi.nlm.nih.gov | |
Description | Data deposited in or computed by PubChem | |
InChI |
InChI=1S/C22H22O10/c1-29-15-5-2-10(6-14(15)24)13-9-30-16-7-11(3-4-12(16)18(13)25)31-22-21(28)20(27)19(26)17(8-23)32-22/h2-7,9,17,19-24,26-28H,8H2,1H3/t17-,19-,20+,21-,22-/m1/s1 | |
Source | PubChem | |
URL | https://pubchem.ncbi.nlm.nih.gov | |
Description | Data deposited in or computed by PubChem | |
InChI Key |
WACBUPFEGWUGPB-MIUGBVLSSA-N | |
Source | PubChem | |
URL | https://pubchem.ncbi.nlm.nih.gov | |
Description | Data deposited in or computed by PubChem | |
Canonical SMILES |
COC1=C(C=C(C=C1)C2=COC3=C(C2=O)C=CC(=C3)OC4C(C(C(C(O4)CO)O)O)O)O | |
Source | PubChem | |
URL | https://pubchem.ncbi.nlm.nih.gov | |
Description | Data deposited in or computed by PubChem | |
Isomeric SMILES |
COC1=C(C=C(C=C1)C2=COC3=C(C2=O)C=CC(=C3)O[C@H]4[C@@H]([C@H]([C@@H]([C@H](O4)CO)O)O)O)O | |
Source | PubChem | |
URL | https://pubchem.ncbi.nlm.nih.gov | |
Description | Data deposited in or computed by PubChem | |
Molecular Formula |
C22H22O10 | |
Source | PubChem | |
URL | https://pubchem.ncbi.nlm.nih.gov | |
Description | Data deposited in or computed by PubChem | |
Molecular Weight |
446.4 g/mol | |
Source | PubChem | |
URL | https://pubchem.ncbi.nlm.nih.gov | |
Description | Data deposited in or computed by PubChem | |
CAS No. |
20633-67-4 | |
Record name | Calycosin 7-o-beta-D-glucoside | |
Source | ChemIDplus | |
URL | https://pubchem.ncbi.nlm.nih.gov/substance/?source=chemidplus&sourceid=0020633674 | |
Description | ChemIDplus is a free, web search system that provides access to the structure and nomenclature authority files used for the identification of chemical substances cited in National Library of Medicine (NLM) databases, including the TOXNET system. | |
Record name | CALYCOSIN 7-O-.BETA.-D-GLUCOSIDE | |
Source | FDA Global Substance Registration System (GSRS) | |
URL | https://gsrs.ncats.nih.gov/ginas/app/beta/substances/247AW3A88C | |
Description | The FDA Global Substance Registration System (GSRS) enables the efficient and accurate exchange of information on what substances are in regulated products. Instead of relying on names, which vary across regulatory domains, countries, and regions, the GSRS knowledge base makes it possible for substances to be defined by standardized, scientific descriptions. | |
Explanation | Unless otherwise noted, the contents of the FDA website (www.fda.gov), both text and graphics, are not copyrighted. They are in the public domain and may be republished, reprinted and otherwise used freely by anyone without the need to obtain permission from FDA. Credit to the U.S. Food and Drug Administration as the source is appreciated but not required. | |
Retrosynthesis Analysis
AI-Powered Synthesis Planning: Our tool employs the Template_relevance Pistachio, Template_relevance Bkms_metabolic, Template_relevance Pistachio_ringbreaker, Template_relevance Reaxys, Template_relevance Reaxys_biocatalysis model, leveraging a vast database of chemical reactions to predict feasible synthetic routes.
One-Step Synthesis Focus: Specifically designed for one-step synthesis, it provides concise and direct routes for your target compounds, streamlining the synthesis process.
Accurate Predictions: Utilizing the extensive PISTACHIO, BKMS_METABOLIC, PISTACHIO_RINGBREAKER, REAXYS, REAXYS_BIOCATALYSIS database, our tool offers high-accuracy predictions, reflecting the latest in chemical research and data.
Strategy Settings
Precursor scoring | Relevance Heuristic |
---|---|
Min. plausibility | 0.01 |
Model | Template_relevance |
Template Set | Pistachio/Bkms_metabolic/Pistachio_ringbreaker/Reaxys/Reaxys_biocatalysis |
Top-N result to add to graph | 6 |
Feasible Synthetic Routes
Q1: What is Calycosin 7-O-glucoside and where is it found?
A1: Calycosin 7-O-glucoside is a flavonoid glycoside found in several plants, notably in the roots of Astragalus membranaceus [], a plant widely used in traditional Chinese medicine.
Q2: What biological activities have been attributed to Calycosin 7-O-glucoside?
A2: Research suggests that Calycosin 7-O-glucoside may play a role in various biological processes. Studies have investigated its potential as an anti-inflammatory agent in the context of Rheumatoid Arthritis, particularly targeting interleukin-6 (IL-6) []. Additionally, it has been explored for its potential to promote nerve function recovery after ischemic stroke, potentially through regulating oxidative stress and apoptosis [, ].
Q3: How does Calycosin 7-O-glucoside interact with biological targets?
A3: While the precise mechanisms of action are still under investigation, research suggests that Calycosin 7-O-glucoside may exert its effects through binding to specific protein targets. For instance, computational studies have suggested its potential to bind to IL-6, suggesting a potential mechanism for its anti-inflammatory effects []. Another study using live HT22 cells combined with affinity chromatography identified Calycosin 7-O-glucoside as a compound with specific binding affinity, further suggesting its interaction with cellular targets [, ].
Q4: Has the pharmacokinetic profile of Calycosin 7-O-glucoside been studied?
A4: Yes, pharmacokinetic studies in mice have detected Calycosin 7-O-glucoside in plasma following oral administration of Radix Astragali extract []. This suggests that it is absorbed into the bloodstream after oral intake.
Q5: What analytical techniques are commonly used to identify and quantify Calycosin 7-O-glucoside?
A5: Various analytical techniques have been employed to identify and quantify Calycosin 7-O-glucoside. These include:
- HPLC-MS/MS: This method has been used for both identification and quantification of Calycosin 7-O-glucoside in various studies, including those investigating its pharmacokinetics and presence in traditional medicine formulations [, , , , , , ].
- UPLC-Q-TOF-MS/MS: This high-resolution mass spectrometry technique has been employed for the characterization and identification of Calycosin 7-O-glucoside in complex mixtures, such as those found in traditional Chinese medicine formulas [].
- UFLC-DAD-Q-TOF-MS/MS: This method combines the separation power of ultra-fast liquid chromatography with the sensitivity and selectivity of tandem mass spectrometry for the identification and analysis of Calycosin 7-O-glucoside [].
- HPLC-PDA: This technique, combining high-performance liquid chromatography with a photodiode array detector, has been utilized for the simultaneous quantification of multiple compounds including Calycosin 7-O-glucoside in traditional Korean medicine formulations [].
- UFLC-IT-TOF–MS/MS and HPLC–DAD: This combination of analytical techniques was used for the identification and quantification of "quality markers" including Calycosin 7-O-glucoside in Tongkang Tablet, highlighting its importance in traditional medicine formulations [].
Q6: Has Calycosin 7-O-glucoside been identified as a "quality marker" in any traditional medicine formulations?
A6: Yes, Calycosin 7-O-glucoside has been identified as a potential "quality marker" in traditional medicine formulations like Tongkang Tablet [], suggesting its significance in contributing to the overall efficacy of these preparations.
Q7: Can Calycosin 7-O-glucoside influence the growth of plants under stress conditions?
A7: Research suggests that the presence of Calycosin 7-O-glucoside may be influenced by environmental factors. One study found that Astragalus mongholicus plants inoculated with Pseudomonas poae strain S61, an endophytic bacterium, showed increased accumulation of Calycosin 7-O-glucoside in their roots when subjected to drought stress []. This indicates a potential role of Calycosin 7-O-glucoside in the plant's response to drought.
試験管内研究製品の免責事項と情報
BenchChemで提示されるすべての記事および製品情報は、情報提供を目的としています。BenchChemで購入可能な製品は、生体外研究のために特別に設計されています。生体外研究は、ラテン語の "in glass" に由来し、生物体の外で行われる実験を指します。これらの製品は医薬品または薬として分類されておらず、FDAから任何の医療状態、病気、または疾患の予防、治療、または治癒のために承認されていません。これらの製品を人間または動物に体内に導入する形態は、法律により厳格に禁止されています。これらのガイドラインに従うことは、研究と実験において法的および倫理的な基準の遵守を確実にするために重要です。