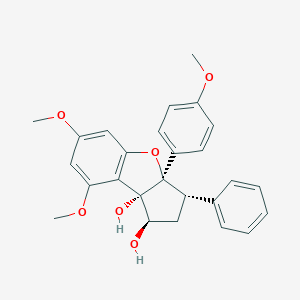
ロカグラオール
概要
説明
Rocaglaol is a substance used for research and development purposes . It is not advised for medicinal, household, or other uses .
Synthesis Analysis
A synthetic analogue of Rocaglaol has been developed . The synthesis process involves the use of a dual-metal-catalyzed asymmetric allylation of benzofuran-3(2H)-one .Molecular Structure Analysis
Rocaglaol is a type of flavagline, which are formed by the cycloaddition of a flavonoid nucleus with a cinnamic acid moiety . It has a molecular formula of C26H26O6 and a molecular weight of 434.48 .Chemical Reactions Analysis
Flavaglines, including Rocaglaol, are formed by the cycloaddition of a flavonoid nucleus with a cinnamic acid moiety . Rocagloic acid is a biosynthetic precursor from which aglafoline- and rocaglamide-type cyclopentabenzofurans can be derived, while those of the rocaglaol-type are the result of decarboxylation .Physical and Chemical Properties Analysis
Rocaglaol is a powder with a molecular formula of C26H26O6 and a molecular weight of 434.48 . It is recommended that stock solutions, once prepared, are stored aliquoted in tightly sealed vials and used within 1 month .科学的研究の応用
タンパク質翻訳の阻害
ロカグラオールとその誘導体であるロカグラートは、タンパク質翻訳を阻害することが報告されている。 これは、タンパク質合成の開始に重要な役割を果たす、eIF4F複合体のRNAヘリカーゼサブユニットであるeIF4Aの活性を調節することによって達成される .
抗腫瘍活性
いくつかのロカグラオール誘導体は、さまざまな腫瘍細胞株に対して良好な細胞毒性を示してきた。 たとえば、Aglaia odorataから単離された8つの誘導体は、0.013〜5.82μMの範囲のIC50値で有意な細胞毒性を示した . さらに、ロカグラオールのC3に修飾を加えた新規誘導体が合成され、3つの腫瘍細胞株(HEL、MDA-MB-231、およびHCT116)に対する抗腫瘍活性がスクリーニングされた .
アポトーシスの誘導
ロカグラオール誘導体は、HCT116細胞でアポトーシスを誘導することも発見されている。 このプロセスは、細胞増殖と生存に関与するMAPKシグナル伝達経路の抑制を介して起こる .
作用機序
Target of Action
Rocaglaol, a highly bioactive flavolignan derived from the Aglaia species, primarily targets the eukaryotic initiation factor (eIF) 4A . This factor is an ATP-dependent DEAD-box RNA helicase, which plays a crucial role in unwinding secondary structures at the 5′-UTRs (untranslated regions) of mRNA to enable binding of the ribosomal pre-initiation complex . Rocaglaol also binds to prohibitins (PHB), which are responsible for regulating important signaling pathways .
Mode of Action
Rocaglaol interacts with its targets by inhibiting protein synthesis. It achieves this by binding to the translation initiation factor eIF4A, thereby preventing the unwinding of secondary structures at the 5′-UTRs of mRNA . This inhibition disrupts the binding of the ribosomal pre-initiation complex, effectively halting protein synthesis .
Biochemical Pathways
The primary biochemical pathway affected by Rocaglaol is the protein synthesis pathway. By inhibiting eIF4A, Rocaglaol disrupts the translation initiation process, leading to a decrease in protein synthesis . This has downstream effects on cellular functions that rely on the production of new proteins. Additionally, Rocaglaol’s interaction with prohibitins can influence various signaling pathways regulated by these proteins .
Pharmacokinetics
This suggests that Rocaglaol can be effectively absorbed and distributed within the body when administered orally .
Result of Action
The inhibition of protein synthesis by Rocaglaol leads to significant molecular and cellular effects. It has been found to induce apoptosis and cell cycle arrest in various human cancer cell lines . Moreover, Rocaglaol can reduce tissue inflammation and neuronal cell death by inhibiting NF-kappa B and AP-1 signaling, resulting in significant neuroprotection in animal models of neurodegeneration .
Action Environment
The action, efficacy, and stability of Rocaglaol can be influenced by various environmental factors It’s worth noting that the effectiveness of Rocaglaol can vary depending on the specific physiological and pathological context within the body. For instance, its anticancer activity can vary significantly among different cancer cell lines .
Safety and Hazards
Rocaglaol is for R&D use only and is not advised for medicinal, household, or other uses . It is recommended to avoid dust formation, breathing mist, gas or vapours, and contact with skin and eye . Use personal protective equipment, wear chemical impermeable gloves, ensure adequate ventilation, remove all sources of ignition, and evacuate personnel to safe areas .
将来の方向性
Recent research has focused on the stereodivergent total synthesis of Rocaglaol . This research has led to the development of a dual-metal-catalyzed asymmetric allylation of benzofuran-3(2H)-one, which has enabled the synthesis of eight stereoisomers of Rocaglaol . This research is expected to contribute to the understanding of the structure-activity relationships of Rocaglaol .
生化学分析
Biochemical Properties
Rocaglaol interacts with various enzymes, proteins, and other biomolecules. It is known to bind to the translation initiation factor eIF4A, an ATP-dependent DEAD-box RNA helicase . This interaction plays a crucial role in its biochemical reactions . The compound also exhibits binding interactions with prohibitins (PHB), which are responsible for regulating important signaling pathways .
Cellular Effects
Rocaglaol has significant effects on various types of cells and cellular processes. It has been found to inhibit cell proliferation and viability in a low nanomolar range . Rocaglaol has shown to induce apoptosis in various tumor cell lines . It also influences cell function by impacting cell signaling pathways, gene expression, and cellular metabolism .
Molecular Mechanism
Rocaglaol exerts its effects at the molecular level through several mechanisms. The primary effect is the inhibition of protein synthesis by binding to the translation initiation factor eIF4A . This binding interaction leads to changes in gene expression and can result in enzyme inhibition or activation .
Temporal Effects in Laboratory Settings
The effects of Rocaglaol change over time in laboratory settings. It has been observed that Rocaglaol exhibits cytotoxic effects against tumor cell lines with IC50 values ranging from 0.007 to 0.095 µmol . Information on the product’s stability, degradation, and any long-term effects on cellular function observed in in vitro or in vivo studies is currently limited.
Dosage Effects in Animal Models
The effects of Rocaglaol vary with different dosages in animal models. A synthetic derivative of Rocaglaol has shown neuroprotective activity in vitro and in animal models of Parkinson’s disease and traumatic brain injury
Metabolic Pathways
Rocaglaol is involved in several metabolic pathways. It is known to inhibit the eukaryotic translation initiation factor 4A (eIF4A), affecting protein translation . Detailed information on the specific metabolic pathways that Rocaglaol is involved in, including any enzymes or cofactors it interacts with, is currently limited.
特性
IUPAC Name |
(1R,3S,3aR,8bS)-6,8-dimethoxy-3a-(4-methoxyphenyl)-3-phenyl-2,3-dihydro-1H-cyclopenta[b][1]benzofuran-1,8b-diol | |
---|---|---|
Source | PubChem | |
URL | https://pubchem.ncbi.nlm.nih.gov | |
Description | Data deposited in or computed by PubChem | |
InChI |
InChI=1S/C26H26O6/c1-29-18-11-9-17(10-12-18)26-20(16-7-5-4-6-8-16)15-23(27)25(26,28)24-21(31-3)13-19(30-2)14-22(24)32-26/h4-14,20,23,27-28H,15H2,1-3H3/t20-,23+,25+,26-/m0/s1 | |
Source | PubChem | |
URL | https://pubchem.ncbi.nlm.nih.gov | |
Description | Data deposited in or computed by PubChem | |
InChI Key |
RRVZOJQBRVGMMK-HCBGRYSISA-N | |
Source | PubChem | |
URL | https://pubchem.ncbi.nlm.nih.gov | |
Description | Data deposited in or computed by PubChem | |
Canonical SMILES |
COC1=CC=C(C=C1)C23C(CC(C2(C4=C(O3)C=C(C=C4OC)OC)O)O)C5=CC=CC=C5 | |
Source | PubChem | |
URL | https://pubchem.ncbi.nlm.nih.gov | |
Description | Data deposited in or computed by PubChem | |
Isomeric SMILES |
COC1=CC=C(C=C1)[C@]23[C@@H](C[C@H]([C@]2(C4=C(O3)C=C(C=C4OC)OC)O)O)C5=CC=CC=C5 | |
Source | PubChem | |
URL | https://pubchem.ncbi.nlm.nih.gov | |
Description | Data deposited in or computed by PubChem | |
Molecular Formula |
C26H26O6 | |
Source | PubChem | |
URL | https://pubchem.ncbi.nlm.nih.gov | |
Description | Data deposited in or computed by PubChem | |
Molecular Weight |
434.5 g/mol | |
Source | PubChem | |
URL | https://pubchem.ncbi.nlm.nih.gov | |
Description | Data deposited in or computed by PubChem | |
Retrosynthesis Analysis
AI-Powered Synthesis Planning: Our tool employs the Template_relevance Pistachio, Template_relevance Bkms_metabolic, Template_relevance Pistachio_ringbreaker, Template_relevance Reaxys, Template_relevance Reaxys_biocatalysis model, leveraging a vast database of chemical reactions to predict feasible synthetic routes.
One-Step Synthesis Focus: Specifically designed for one-step synthesis, it provides concise and direct routes for your target compounds, streamlining the synthesis process.
Accurate Predictions: Utilizing the extensive PISTACHIO, BKMS_METABOLIC, PISTACHIO_RINGBREAKER, REAXYS, REAXYS_BIOCATALYSIS database, our tool offers high-accuracy predictions, reflecting the latest in chemical research and data.
Strategy Settings
Precursor scoring | Relevance Heuristic |
---|---|
Min. plausibility | 0.01 |
Model | Template_relevance |
Template Set | Pistachio/Bkms_metabolic/Pistachio_ringbreaker/Reaxys/Reaxys_biocatalysis |
Top-N result to add to graph | 6 |
Feasible Synthetic Routes
試験管内研究製品の免責事項と情報
BenchChemで提示されるすべての記事および製品情報は、情報提供を目的としています。BenchChemで購入可能な製品は、生体外研究のために特別に設計されています。生体外研究は、ラテン語の "in glass" に由来し、生物体の外で行われる実験を指します。これらの製品は医薬品または薬として分類されておらず、FDAから任何の医療状態、病気、または疾患の予防、治療、または治癒のために承認されていません。これらの製品を人間または動物に体内に導入する形態は、法律により厳格に禁止されています。これらのガイドラインに従うことは、研究と実験において法的および倫理的な基準の遵守を確実にするために重要です。