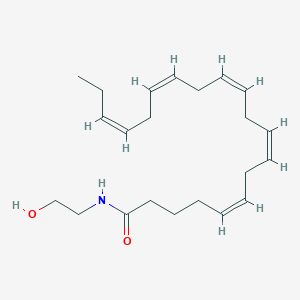
エイコサペンタエノイルエタノールアミド
説明
Eicosapentaenoyl Ethanolamide is a bioactive lipid belonging to the N-acylethanolamine family. It is derived from eicosapentaenoic acid, an omega-3 fatty acid. This compound is known for its role as a cannabinoid receptor agonist, interacting with both CB1 and CB2 receptors. It has been studied for its potential anti-inflammatory, anti-proliferative, and metabolic signaling properties .
科学的研究の応用
Chemistry:
- Used as a model compound to study the behavior of N-acylethanolamines in different chemical reactions.
Biology:
- Investigated for its role in cell signaling and metabolic regulation. It has been shown to influence cell proliferation and apoptosis in various cell lines .
Medicine:
- Potential therapeutic agent for inflammatory diseases due to its anti-inflammatory properties.
- Studied for its anti-cancer effects, particularly in prostate cancer cell lines .
Industry:
- Used in the development of nutraceuticals and functional foods due to its omega-3 fatty acid content.
作用機序
エイコサペンタエノイルエタノールアミドは、主にカンナビノイド受容体CB1とCB2の活性化を通じて作用します。これらの受容体は、痛み、食欲、免疫応答などのさまざまな生理学的プロセスを調節する上で重要な役割を果たすエンドカンナビノイドシステムの一部です。 これらの受容体に結合することにより、エイコサペンタエノイルエタノールアミドは、炎症、細胞増殖、代謝に関与するシグナル伝達経路を調節できます .
類似化合物:
アラキドノイルエタノールアミド(アナンダミド): カンナビノイド受容体に対する内因性リガンドとして作用する別のN-アシルエタノールアミン。痛み調節と神経保護に関与しています。
ドコサヘキサエノイルエタノールアミド: ドコサヘキサエン酸から誘導されたこの化合物は、カンナビノイド受容体にも作用し、神経保護作用について研究されています。
独自性: エイコサペンタエノイルエタノールアミドは、他のN-アシルエタノールアミンとは異なる生物学的活性を付与する特定の脂肪酸組成により、ユニークです。 代謝シグナル伝達と寿命調節、特に線虫などのモデル生物における役割は、類似の化合物とは異なります .
生化学分析
Biochemical Properties
Eicosapentaenoyl Ethanolamide plays a crucial role in biochemical reactions by acting as a metabolic signal. It interacts with several enzymes, proteins, and other biomolecules. One of its primary interactions is with cannabinoid receptors CB1 and CB2, where it acts as an agonist . These receptors are involved in various physiological processes, including pain modulation, appetite regulation, and immune response. Additionally, Eicosapentaenoyl Ethanolamide inhibits the dietary restriction-induced lifespan extension in wild-type animals and suppresses lifespan extension in TOR pathway mutants . It also induces cell death in prostate cancer cell lines LNCaP and PC3 .
Cellular Effects
Eicosapentaenoyl Ethanolamide exerts significant effects on various cell types and cellular processes. In psoriatic keratinocytes, it decreases proliferation and reduces inflammatory features . This compound influences cell function by modulating cell signaling pathways, gene expression, and cellular metabolism. For instance, Eicosapentaenoyl Ethanolamide has been shown to inhibit the proliferation of psoriatic keratinocytes by interacting with CB1 receptors . It also affects the expression of specific proteins involved in cell differentiation and inflammation, such as keratin 17 and psoriasin .
Molecular Mechanism
The molecular mechanism of Eicosapentaenoyl Ethanolamide involves its interaction with cannabinoid receptors CB1 and CB2, where it acts as an agonist . This interaction leads to the activation of downstream signaling pathways that regulate various physiological processes. Additionally, Eicosapentaenoyl Ethanolamide acts as a metabolic signal that couples nutrient availability with growth and lifespan . It inhibits the dietary restriction-induced lifespan extension by modulating the TOR pathway . Furthermore, Eicosapentaenoyl Ethanolamide induces cell death in prostate cancer cells through both cannabinoid receptor-dependent and independent mechanisms .
Temporal Effects in Laboratory Settings
In laboratory settings, the effects of Eicosapentaenoyl Ethanolamide can change over time. Studies have shown that it can inhibit the dietary restriction-induced lifespan extension in wild-type animals and suppress lifespan extension in TOR pathway mutants . Additionally, Eicosapentaenoyl Ethanolamide has been found to decrease the proliferation of psoriatic keratinocytes over a period of 7 days . The stability and degradation of Eicosapentaenoyl Ethanolamide in laboratory settings are crucial factors that influence its long-term effects on cellular function.
Dosage Effects in Animal Models
The effects of Eicosapentaenoyl Ethanolamide vary with different dosages in animal models. In Caenorhabditis elegans, dietary supplementation with Eicosapentaenoyl Ethanolamide inhibits dietary restriction-induced lifespan extension in wild-type worms and suppresses lifespan extension in TOR pathway mutants . The compound’s dosage-dependent effects on lifespan and metabolic processes highlight its potential therapeutic applications. High doses of Eicosapentaenoyl Ethanolamide may also lead to toxic or adverse effects, which need to be carefully evaluated in animal studies.
Metabolic Pathways
Eicosapentaenoyl Ethanolamide is involved in several metabolic pathways. It is produced endogenously from eicosapentaenoic acid and serves as a metabolic signal that couples nutrient availability with growth and lifespan . The compound is metabolized by enzymes such as fatty acid amide hydrolase (FAAH) and N-acylethanolamine-hydrolyzing acid amidase (NAAA) . These enzymes play a crucial role in regulating the levels of Eicosapentaenoyl Ethanolamide and its biological effects.
Transport and Distribution
Eicosapentaenoyl Ethanolamide is transported and distributed within cells and tissues through various mechanisms. It interacts with transporters and binding proteins that facilitate its localization and accumulation in specific cellular compartments . For instance, in cerebrospinal fluid, Eicosapentaenoyl Ethanolamide has been detected at significant levels, indicating its role in modulating inflammation and other physiological processes in the central nervous system .
Subcellular Localization
The subcellular localization of Eicosapentaenoyl Ethanolamide is essential for its activity and function. It is believed to localize in specific cellular compartments, where it interacts with target proteins and receptors . The compound’s localization is influenced by targeting signals and post-translational modifications that direct it to specific organelles. For example, in psoriatic keratinocytes, Eicosapentaenoyl Ethanolamide’s effects on cell proliferation and inflammation are mediated through its interaction with CB1 receptors .
準備方法
合成経路および反応条件: エイコサペンタエノイルエタノールアミドは、エイコサペンタエン酸とエタノールアミンをアミド化することにより合成できます。反応は通常、N,N'-ジシクロヘキシルカルボジイミド(DCC)や4-ジメチルアミノピリジン(DMAP)などのカップリング剤を使用し、アミド結合の形成を促進します。 反応は通常、室温でジクロロメタンなどの有機溶媒中で行われます .
工業生産方法: エイコサペンタエノイルエタノールアミドの工業生産には、リパーゼを使用してエイコサペンタエン酸とエタノールアミンの反応を触媒する酵素合成が含まれる場合があります。 この方法は、穏やかな反応条件と高い特異性により、より高い収率とより少ない副生成物を達成できるため、有利です .
反応の種類:
酸化: エイコサペンタエノイルエタノールアミドは、特にエイコサペンタエン酸部分に存在する二重結合で、酸化反応を起こす可能性があります。一般的な酸化剤には、過マンガン酸カリウムやオゾンなどがあります。
還元: エイコサペンタエノイルエタノールアミドの還元は、パラジウム炭素などの水素化触媒を使用して行うことができ、二重結合を単結合に還元します。
置換: エタノールアミン中のヒドロキシル基は置換反応に関与し、使用される試薬に応じてさまざまな誘導体を形成できます。
一般的な試薬と条件:
酸化: 水溶液中の過マンガン酸カリウム。
還元: パラジウム炭素触媒を使用した水素ガス。
置換: トリエチルアミンなどの塩基の存在下でのハロアルカンまたはアシルクロリド。
形成される主要な生成物:
酸化: エポキシドおよびヒドロキシル化誘導体。
還元: 飽和エイコサペンタエノイルエタノールアミド。
置換: N-置換エタノールアミド。
4. 科学研究アプリケーション
化学:
- さまざまな化学反応におけるN-アシルエタノールアミンの挙動を研究するためのモデル化合物として使用されます。
生物学:
- 細胞シグナル伝達と代謝調節における役割について調査されています。 さまざまな細胞株における細胞増殖とアポトーシスに影響を与えることが示されています .
医学:
- 抗炎症作用により、炎症性疾患の潜在的な治療薬です。
- 特に前立腺がん細胞株における抗がん作用について研究されています .
産業:
- オメガ3脂肪酸含有量のために、ニュートラシューティカルや機能性食品の開発に使用されています。
類似化合物との比較
Arachidonoyl Ethanolamide (Anandamide): Another N-acylethanolamine that acts as an endogenous ligand for cannabinoid receptors. It is involved in pain modulation and neuroprotection.
Docosahexaenoyl Ethanolamide: Derived from docosahexaenoic acid, this compound also interacts with cannabinoid receptors and has been studied for its neuroprotective effects.
Uniqueness: Eicosapentaenoyl Ethanolamide is unique due to its specific fatty acid composition, which imparts distinct biological activities compared to other N-acylethanolamines. Its role in metabolic signaling and lifespan regulation, particularly in model organisms like Caenorhabditis elegans, sets it apart from similar compounds .
特性
IUPAC Name |
(5Z,8Z,11Z,14Z,17Z)-N-(2-hydroxyethyl)icosa-5,8,11,14,17-pentaenamide | |
---|---|---|
Source | PubChem | |
URL | https://pubchem.ncbi.nlm.nih.gov | |
Description | Data deposited in or computed by PubChem | |
InChI |
InChI=1S/C22H35NO2/c1-2-3-4-5-6-7-8-9-10-11-12-13-14-15-16-17-18-19-22(25)23-20-21-24/h3-4,6-7,9-10,12-13,15-16,24H,2,5,8,11,14,17-21H2,1H3,(H,23,25)/b4-3-,7-6-,10-9-,13-12-,16-15- | |
Source | PubChem | |
URL | https://pubchem.ncbi.nlm.nih.gov | |
Description | Data deposited in or computed by PubChem | |
InChI Key |
OVKKNJPJQKTXIT-JLNKQSITSA-N | |
Source | PubChem | |
URL | https://pubchem.ncbi.nlm.nih.gov | |
Description | Data deposited in or computed by PubChem | |
Canonical SMILES |
CCC=CCC=CCC=CCC=CCC=CCCCC(=O)NCCO | |
Source | PubChem | |
URL | https://pubchem.ncbi.nlm.nih.gov | |
Description | Data deposited in or computed by PubChem | |
Isomeric SMILES |
CC/C=C\C/C=C\C/C=C\C/C=C\C/C=C\CCCC(=O)NCCO | |
Source | PubChem | |
URL | https://pubchem.ncbi.nlm.nih.gov | |
Description | Data deposited in or computed by PubChem | |
Molecular Formula |
C22H35NO2 | |
Source | PubChem | |
URL | https://pubchem.ncbi.nlm.nih.gov | |
Description | Data deposited in or computed by PubChem | |
DSSTOX Substance ID |
DTXSID901153302 | |
Record name | (5Z,8Z,11Z,14Z,17Z)-N-(2-Hydroxyethyl)-5,8,11,14,17-eicosapentaenamide | |
Source | EPA DSSTox | |
URL | https://comptox.epa.gov/dashboard/DTXSID901153302 | |
Description | DSSTox provides a high quality public chemistry resource for supporting improved predictive toxicology. | |
Molecular Weight |
345.5 g/mol | |
Source | PubChem | |
URL | https://pubchem.ncbi.nlm.nih.gov | |
Description | Data deposited in or computed by PubChem | |
Physical Description |
Solid | |
Record name | Eicosapentaenoyl Ethanolamide | |
Source | Human Metabolome Database (HMDB) | |
URL | http://www.hmdb.ca/metabolites/HMDB0013649 | |
Description | The Human Metabolome Database (HMDB) is a freely available electronic database containing detailed information about small molecule metabolites found in the human body. | |
Explanation | HMDB is offered to the public as a freely available resource. Use and re-distribution of the data, in whole or in part, for commercial purposes requires explicit permission of the authors and explicit acknowledgment of the source material (HMDB) and the original publication (see the HMDB citing page). We ask that users who download significant portions of the database cite the HMDB paper in any resulting publications. | |
CAS No. |
109001-03-8 | |
Record name | (5Z,8Z,11Z,14Z,17Z)-N-(2-Hydroxyethyl)-5,8,11,14,17-eicosapentaenamide | |
Source | CAS Common Chemistry | |
URL | https://commonchemistry.cas.org/detail?cas_rn=109001-03-8 | |
Description | CAS Common Chemistry is an open community resource for accessing chemical information. Nearly 500,000 chemical substances from CAS REGISTRY cover areas of community interest, including common and frequently regulated chemicals, and those relevant to high school and undergraduate chemistry classes. This chemical information, curated by our expert scientists, is provided in alignment with our mission as a division of the American Chemical Society. | |
Explanation | The data from CAS Common Chemistry is provided under a CC-BY-NC 4.0 license, unless otherwise stated. | |
Record name | (5Z,8Z,11Z,14Z,17Z)-N-(2-Hydroxyethyl)-5,8,11,14,17-eicosapentaenamide | |
Source | EPA DSSTox | |
URL | https://comptox.epa.gov/dashboard/DTXSID901153302 | |
Description | DSSTox provides a high quality public chemistry resource for supporting improved predictive toxicology. | |
Record name | Eicosapentaenoyl Ethanolamide | |
Source | Human Metabolome Database (HMDB) | |
URL | http://www.hmdb.ca/metabolites/HMDB0013649 | |
Description | The Human Metabolome Database (HMDB) is a freely available electronic database containing detailed information about small molecule metabolites found in the human body. | |
Explanation | HMDB is offered to the public as a freely available resource. Use and re-distribution of the data, in whole or in part, for commercial purposes requires explicit permission of the authors and explicit acknowledgment of the source material (HMDB) and the original publication (see the HMDB citing page). We ask that users who download significant portions of the database cite the HMDB paper in any resulting publications. | |
Retrosynthesis Analysis
AI-Powered Synthesis Planning: Our tool employs the Template_relevance Pistachio, Template_relevance Bkms_metabolic, Template_relevance Pistachio_ringbreaker, Template_relevance Reaxys, Template_relevance Reaxys_biocatalysis model, leveraging a vast database of chemical reactions to predict feasible synthetic routes.
One-Step Synthesis Focus: Specifically designed for one-step synthesis, it provides concise and direct routes for your target compounds, streamlining the synthesis process.
Accurate Predictions: Utilizing the extensive PISTACHIO, BKMS_METABOLIC, PISTACHIO_RINGBREAKER, REAXYS, REAXYS_BIOCATALYSIS database, our tool offers high-accuracy predictions, reflecting the latest in chemical research and data.
Strategy Settings
Precursor scoring | Relevance Heuristic |
---|---|
Min. plausibility | 0.01 |
Model | Template_relevance |
Template Set | Pistachio/Bkms_metabolic/Pistachio_ringbreaker/Reaxys/Reaxys_biocatalysis |
Top-N result to add to graph | 6 |
Feasible Synthetic Routes
試験管内研究製品の免責事項と情報
BenchChemで提示されるすべての記事および製品情報は、情報提供を目的としています。BenchChemで購入可能な製品は、生体外研究のために特別に設計されています。生体外研究は、ラテン語の "in glass" に由来し、生物体の外で行われる実験を指します。これらの製品は医薬品または薬として分類されておらず、FDAから任何の医療状態、病気、または疾患の予防、治療、または治癒のために承認されていません。これらの製品を人間または動物に体内に導入する形態は、法律により厳格に禁止されています。これらのガイドラインに従うことは、研究と実験において法的および倫理的な基準の遵守を確実にするために重要です。