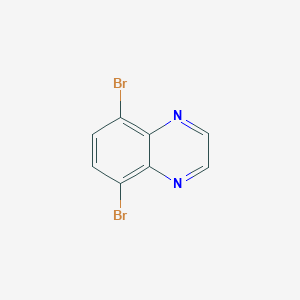
5,8-ジブロモキノキサリン
概要
説明
5,8-Dibromoquinoxaline is a halogenated heterocyclic compound with the molecular formula C8H4Br2N2 and a molecular weight of 287.94 g/mol It is characterized by the presence of two bromine atoms at the 5 and 8 positions of the quinoxaline ring
科学的研究の応用
5,8-Dibromoquinoxaline has a wide range of applications in scientific research, including:
Chemistry: It is used as a building block for the synthesis of various heterocyclic compounds and polymers.
Biology: It serves as a precursor for the development of biologically active molecules with potential therapeutic applications.
Medicine: Research is ongoing to explore its potential as an anticancer and antimicrobial agent.
準備方法
Synthetic Routes and Reaction Conditions: 5,8-Dibromoquinoxaline can be synthesized through the bromination of quinoxaline. The general procedure involves adding bromine dropwise to a magnetically stirred refluxing solution of quinoxaline in an appropriate solvent, such as acetonitrile . The reaction mixture is heated at reflux temperature for a specified period, typically around 25 hours, under an inert atmosphere. The reaction is monitored using thin-layer chromatography or nuclear magnetic resonance spectroscopy. After completion, the mixture is cooled to room temperature, and the solvent is removed under reduced pressure. The crude product is then purified using column chromatography .
Industrial Production Methods: Industrial production of 5,8-Dibromoquinoxaline follows similar synthetic routes but on a larger scale. The process involves the use of high-temperature bromination techniques and efficient purification methods to ensure high yield and purity of the final product .
化学反応の分析
Types of Reactions: 5,8-Dibromoquinoxaline undergoes various chemical reactions, including:
Substitution Reactions: The bromine atoms can be substituted with other functional groups through nucleophilic substitution reactions.
Oxidation and Reduction Reactions: The quinoxaline ring can undergo oxidation and reduction under specific conditions.
Common Reagents and Conditions:
Substitution Reactions: Common reagents include nucleophiles such as amines, thiols, and alkoxides. The reactions are typically carried out in polar solvents like dimethylformamide or dimethyl sulfoxide at elevated temperatures.
Oxidation and Reduction Reactions: Oxidizing agents such as potassium permanganate or reducing agents like sodium borohydride can be used under controlled conditions.
Major Products: The major products formed from these reactions depend on the specific reagents and conditions used. For example, nucleophilic substitution with amines can yield aminoquinoxalines, while oxidation can produce quinoxaline dioxides .
作用機序
The mechanism of action of 5,8-Dibromoquinoxaline involves its interaction with specific molecular targets and pathways. The bromine atoms at the 5 and 8 positions enhance its reactivity, allowing it to participate in various chemical reactions. In biological systems, it can interact with enzymes and receptors, potentially inhibiting their activity and leading to therapeutic effects .
類似化合物との比較
- 2,6-Dichloroquinoxaline
- 2,3-Dichloroquinoxaline
- 2,5-Dibromohydroquinone
Comparison: Compared to other halogenated quinoxalines, it exhibits distinct properties that make it suitable for specialized applications in materials science and medicinal chemistry .
生物活性
5,8-Dibromoquinoxaline is a synthetic compound that has garnered attention for its diverse biological activities. This article explores its pharmacological properties, mechanisms of action, and potential therapeutic applications based on recent research findings.
5,8-Dibromoquinoxaline (CHBrN) is a dibrominated derivative of quinoxaline. The structure consists of a fused bicyclic system containing nitrogen atoms, which contributes to its biological activity. The compound is characterized by the presence of two bromine atoms at the 5 and 8 positions of the quinoxaline ring, enhancing its reactivity and interaction with biological targets.
Antiproliferative Effects
Research has demonstrated that 5,8-dibromoquinoxaline exhibits significant antiproliferative activity against various cell lines. A study evaluated its effects on rat aortic smooth muscle cells (RAoSMCs), revealing that it inhibits cell proliferation through modulation of the extracellular signal-regulated kinase (ERK) signaling pathway. This mechanism suggests potential applications in treating vascular diseases where smooth muscle cell proliferation is a contributing factor .
Antimicrobial Properties
The compound has also shown promising antimicrobial properties. It was found to possess bacteriostatic and fungistatic activities, particularly effective against certain strains of bacteria and fungi. These findings indicate its potential use as an antibacterial or antifungal agent, although specific mechanisms remain to be fully elucidated .
Study 1: Inhibition of Smooth Muscle Cell Proliferation
A detailed investigation into the antiproliferative effects of quinoxaline derivatives, including 5,8-dibromoquinoxaline, highlighted its ability to inhibit RAoSMC proliferation. The study utilized various concentrations of the compound and assessed cell viability through MTT assays. The results indicated a dose-dependent response, with significant inhibition observed at higher concentrations.
Concentration (µM) | % Inhibition |
---|---|
5 | 20 |
10 | 40 |
25 | 70 |
50 | 90 |
This data underscores the compound's potential as a therapeutic agent in conditions characterized by excessive smooth muscle proliferation .
Study 2: Antimicrobial Activity Assessment
In another study focusing on antimicrobial activity, 5,8-dibromoquinoxaline was tested against various bacterial strains using disc diffusion methods. The results indicated that the compound displayed notable inhibitory zones against Gram-positive bacteria such as Staphylococcus aureus and Gram-negative bacteria like Escherichia coli.
Bacterial Strain | Inhibition Zone (mm) |
---|---|
Staphylococcus aureus | 15 |
Escherichia coli | 12 |
Candida albicans | 14 |
These findings suggest that 5,8-dibromoquinoxaline may serve as a potential lead compound for developing new antimicrobial agents .
The biological activity of 5,8-dibromoquinoxaline can be attributed to several mechanisms:
- Inhibition of Kinase Activity : The modulation of ERK signaling pathways indicates that the compound may interfere with kinase activities crucial for cell proliferation.
- Intercalation with DNA : Similar compounds have been shown to intercalate with DNA, potentially leading to cytotoxic effects in rapidly dividing cells.
- Reactive Oxygen Species (ROS) Generation : Some studies suggest that quinoxaline derivatives can induce oxidative stress in cells, contributing to their antiproliferative effects.
特性
IUPAC Name |
5,8-dibromoquinoxaline | |
---|---|---|
Source | PubChem | |
URL | https://pubchem.ncbi.nlm.nih.gov | |
Description | Data deposited in or computed by PubChem | |
InChI |
InChI=1S/C8H4Br2N2/c9-5-1-2-6(10)8-7(5)11-3-4-12-8/h1-4H | |
Source | PubChem | |
URL | https://pubchem.ncbi.nlm.nih.gov | |
Description | Data deposited in or computed by PubChem | |
InChI Key |
ZPZBXKVJNVNNET-UHFFFAOYSA-N | |
Source | PubChem | |
URL | https://pubchem.ncbi.nlm.nih.gov | |
Description | Data deposited in or computed by PubChem | |
Canonical SMILES |
C1=CC(=C2C(=C1Br)N=CC=N2)Br | |
Source | PubChem | |
URL | https://pubchem.ncbi.nlm.nih.gov | |
Description | Data deposited in or computed by PubChem | |
Molecular Formula |
C8H4Br2N2 | |
Source | PubChem | |
URL | https://pubchem.ncbi.nlm.nih.gov | |
Description | Data deposited in or computed by PubChem | |
DSSTOX Substance ID |
DTXSID60467892 | |
Record name | 5,8-dibromoquinoxaline | |
Source | EPA DSSTox | |
URL | https://comptox.epa.gov/dashboard/DTXSID60467892 | |
Description | DSSTox provides a high quality public chemistry resource for supporting improved predictive toxicology. | |
Molecular Weight |
287.94 g/mol | |
Source | PubChem | |
URL | https://pubchem.ncbi.nlm.nih.gov | |
Description | Data deposited in or computed by PubChem | |
CAS No. |
148231-12-3 | |
Record name | 5,8-dibromoquinoxaline | |
Source | EPA DSSTox | |
URL | https://comptox.epa.gov/dashboard/DTXSID60467892 | |
Description | DSSTox provides a high quality public chemistry resource for supporting improved predictive toxicology. | |
Record name | 5,8-Dibromoquinoxaline | |
Source | European Chemicals Agency (ECHA) | |
URL | https://echa.europa.eu/information-on-chemicals | |
Description | The European Chemicals Agency (ECHA) is an agency of the European Union which is the driving force among regulatory authorities in implementing the EU's groundbreaking chemicals legislation for the benefit of human health and the environment as well as for innovation and competitiveness. | |
Explanation | Use of the information, documents and data from the ECHA website is subject to the terms and conditions of this Legal Notice, and subject to other binding limitations provided for under applicable law, the information, documents and data made available on the ECHA website may be reproduced, distributed and/or used, totally or in part, for non-commercial purposes provided that ECHA is acknowledged as the source: "Source: European Chemicals Agency, http://echa.europa.eu/". Such acknowledgement must be included in each copy of the material. ECHA permits and encourages organisations and individuals to create links to the ECHA website under the following cumulative conditions: Links can only be made to webpages that provide a link to the Legal Notice page. | |
Synthesis routes and methods
Procedure details
Retrosynthesis Analysis
AI-Powered Synthesis Planning: Our tool employs the Template_relevance Pistachio, Template_relevance Bkms_metabolic, Template_relevance Pistachio_ringbreaker, Template_relevance Reaxys, Template_relevance Reaxys_biocatalysis model, leveraging a vast database of chemical reactions to predict feasible synthetic routes.
One-Step Synthesis Focus: Specifically designed for one-step synthesis, it provides concise and direct routes for your target compounds, streamlining the synthesis process.
Accurate Predictions: Utilizing the extensive PISTACHIO, BKMS_METABOLIC, PISTACHIO_RINGBREAKER, REAXYS, REAXYS_BIOCATALYSIS database, our tool offers high-accuracy predictions, reflecting the latest in chemical research and data.
Strategy Settings
Precursor scoring | Relevance Heuristic |
---|---|
Min. plausibility | 0.01 |
Model | Template_relevance |
Template Set | Pistachio/Bkms_metabolic/Pistachio_ringbreaker/Reaxys/Reaxys_biocatalysis |
Top-N result to add to graph | 6 |
Feasible Synthetic Routes
Q1: What makes 5,8-Dibromoquinoxaline suitable for use in conjugated polymers?
A1: 5,8-Dibromoquinoxaline acts as an electron-accepting unit due to the electron-withdrawing nature of the quinoxaline moiety. When copolymerized with electron-donating monomers, it forms D-A conjugated polymers. [, , , , , ] These polymers exhibit intramolecular charge transfer, leading to desirable properties like narrow band gaps and broad absorption in the visible region, crucial for applications like organic photovoltaics and light-emitting diodes. [, , , ]
Q2: How does modifying the donor unit in a copolymer containing 5,8-Dibromoquinoxaline affect the polymer's properties?
A2: Research shows that incorporating different donor units alongside 5,8-Dibromoquinoxaline significantly impacts the polymer's properties. For instance, using thiophene units instead of benzodithiophene led to a more planar polymer backbone in PTQTS, enhancing intermolecular π-stacking and ultimately improving photovoltaic performance. [] This highlights how structural modifications tune the polymer's electronic and optical characteristics.
Q3: Can you elaborate on the impact of structural modifications on the absorption properties of these polymers?
A3: Studies show a direct correlation between the structure of the acceptor unit and the polymer's absorption properties. For example, increasing the electron-withdrawing ability of the co-monomer by using 5,8-dibromo-2,3-diphenylquinoxaline or 10,13-dibromodibenzo[a,c]phenazine instead of 5,8-dibromoquinoxaline resulted in a redshift in the absorption spectra of the resulting polymers. [] This modification allows for better harvesting of light in the visible region, crucial for solar cell applications.
Q4: Beyond organic photovoltaics, are there other potential applications for these D-A polymers?
A4: Yes, these polymers exhibit promising electrochromic properties. Research on polymers incorporating 2,3-di(2-pyridyl)-5,8-dibromoquinoxaline as the acceptor unit demonstrated their ability to switch between different colors upon electrochemical oxidation and reduction. [] These properties, coupled with high optical contrasts in the near-infrared region and fast switching times, make them suitable candidates for applications like smart windows and displays.
試験管内研究製品の免責事項と情報
BenchChemで提示されるすべての記事および製品情報は、情報提供を目的としています。BenchChemで購入可能な製品は、生体外研究のために特別に設計されています。生体外研究は、ラテン語の "in glass" に由来し、生物体の外で行われる実験を指します。これらの製品は医薬品または薬として分類されておらず、FDAから任何の医療状態、病気、または疾患の予防、治療、または治癒のために承認されていません。これらの製品を人間または動物に体内に導入する形態は、法律により厳格に禁止されています。これらのガイドラインに従うことは、研究と実験において法的および倫理的な基準の遵守を確実にするために重要です。