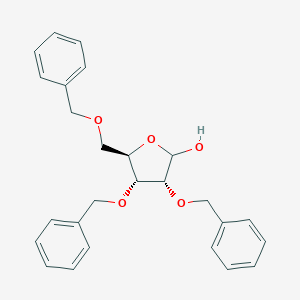
2,3,5-トリ-O-ベンジル-D-リボフラノース
- 専門家チームからの見積もりを受け取るには、QUICK INQUIRYをクリックしてください。
- 品質商品を競争力のある価格で提供し、研究に集中できます。
概要
説明
2,3,5-Tri-O-benzyl-D-ribofuranose is a derivative of ribose, a naturally occurring sugar. This compound is characterized by the presence of three benzyl groups attached to the ribofuranose ring. It has the molecular formula C26H28O5 and a molecular weight of 420.497 Da . The compound is often used in organic synthesis, particularly in the preparation of nucleoside analogs and other complex molecules.
科学的研究の応用
2,3,5-Tri-O-benzyl-D-ribofuranose has several applications in scientific research:
Chemistry: It is used as an intermediate in the synthesis of nucleoside analogs and other complex organic molecules.
Biology: The compound is used in the study of carbohydrate metabolism and enzyme interactions.
Medicine: It serves as a precursor in the synthesis of antiviral and anticancer agents.
Industry: The compound is used in the production of pharmaceuticals and fine chemicals.
作用機序
Target of Action
The primary target of 2,3,5-Tri-O-benzyl-D-ribofuranose is the chitin synthase (CHS) enzyme . This enzyme plays a crucial role in the synthesis of chitin, a key component of the cell walls of fungi .
Mode of Action
2,3,5-Tri-O-benzyl-D-ribofuranose acts as an inhibitor of the chitin synthase enzyme . By binding to this enzyme, it prevents the normal synthesis of chitin, thereby disrupting the structural integrity of the fungal cell wall .
Biochemical Pathways
The inhibition of chitin synthase by 2,3,5-Tri-O-benzyl-D-ribofuranose affects the chitin biosynthesis pathway . This disruption leads to a decrease in chitin production, which in turn weakens the cell wall of the fungus and inhibits its growth .
Result of Action
The inhibition of chitin synthase by 2,3,5-Tri-O-benzyl-D-ribofuranose results in antifungal activity . Specifically, it has been shown to inhibit the growth of the fungus Botrytis cinerea .
Action Environment
The action, efficacy, and stability of 2,3,5-Tri-O-benzyl-D-ribofuranose can be influenced by various environmental factors. For instance, its solubility and therefore its bioavailability and efficacy can be affected by the pH and temperature of the environment . Additionally, its stability could be impacted by exposure to light, heat, and moisture .
生化学分析
Biochemical Properties
2,3,5-Tri-O-benzyl-D-ribofuranose is an effective inhibitor of Botrytis cinerea chitin synthase (CHS), with an IC50 value of 1.8 μM . It exhibits antifungal activity and is able to inhibit the B. cinerea BD90 strain, with a MIC value of 190 μM .
Cellular Effects
The antifungal activity of 2,3,5-Tri-O-benzyl-D-ribofuranose suggests that it can affect various types of cells, particularly fungal cells. By inhibiting the chitin synthase enzyme, it disrupts the formation of chitin, a key component of the fungal cell wall . This can lead to the disruption of cellular processes and ultimately cell death.
Molecular Mechanism
The molecular mechanism of 2,3,5-Tri-O-benzyl-D-ribofuranose involves the inhibition of the enzyme chitin synthase. This enzyme is crucial for the synthesis of chitin, a major component of the cell walls of fungi . By inhibiting this enzyme, 2,3,5-Tri-O-benzyl-D-ribofuranose disrupts the integrity of the fungal cell wall, leading to cell death .
Temporal Effects in Laboratory Settings
It is known that the compound is stable under normal temperatures and pressures .
準備方法
Synthetic Routes and Reaction Conditions
The synthesis of 2,3,5-Tri-O-benzyl-D-ribofuranose typically involves the protection of the hydroxyl groups of D-ribose with benzyl groups. One common method involves the use of benzyl chloride in the presence of a base such as sodium hydride or potassium carbonate. The reaction is usually carried out in an aprotic solvent like dimethylformamide (DMF) or tetrahydrofuran (THF) at elevated temperatures .
Industrial Production Methods
Industrial production of 2,3,5-Tri-O-benzyl-D-ribofuranose follows similar synthetic routes but on a larger scale. The process involves the use of large reactors and precise control of reaction conditions to ensure high yield and purity. The product is typically purified by recrystallization or chromatography .
化学反応の分析
Types of Reactions
2,3,5-Tri-O-benzyl-D-ribofuranose undergoes various chemical reactions, including:
Oxidation: The compound can be oxidized to form corresponding aldehydes or carboxylic acids.
Reduction: Reduction reactions can convert the compound into alcohols or other reduced forms.
Substitution: The benzyl groups can be substituted with other functional groups through nucleophilic substitution reactions.
Common Reagents and Conditions
Oxidation: Common oxidizing agents include potassium permanganate (KMnO4) and chromium trioxide (CrO3).
Reduction: Reducing agents such as lithium aluminum hydride (LiAlH4) or sodium borohydride (NaBH4) are often used.
Substitution: Nucleophiles like sodium methoxide (NaOMe) or potassium tert-butoxide (KOtBu) can be used for substitution reactions.
Major Products Formed
The major products formed from these reactions depend on the specific conditions and reagents used. For example, oxidation can yield benzyl aldehydes or carboxylic acids, while reduction can produce benzyl alcohols .
類似化合物との比較
Similar Compounds
1-O-Acetyl-2,3,5-tri-O-benzoyl-β-D-ribofuranose: This compound is also a ribose derivative with benzoyl groups instead of benzyl groups.
2,3,5-Tri-O-benzyl-β-D-arabinofuranose: Similar to 2,3,5-Tri-O-benzyl-D-ribofuranose but derived from arabinose instead of ribose.
Uniqueness
2,3,5-Tri-O-benzyl-D-ribofuranose is unique due to its specific substitution pattern and the presence of benzyl groups, which provide distinct steric and electronic properties. These characteristics make it particularly useful in the synthesis of nucleoside analogs and other complex molecules .
特性
CAS番号 |
16838-89-4 |
---|---|
分子式 |
C26H28O5 |
分子量 |
420.5 g/mol |
IUPAC名 |
(4S,5S)-3,4-bis(phenylmethoxy)-5-(phenylmethoxymethyl)oxolan-2-ol |
InChI |
InChI=1S/C26H28O5/c27-26-25(30-18-22-14-8-3-9-15-22)24(29-17-21-12-6-2-7-13-21)23(31-26)19-28-16-20-10-4-1-5-11-20/h1-15,23-27H,16-19H2/t23-,24-,25?,26?/m0/s1 |
InChIキー |
NAQUAXSCBJPECG-ZCCOPBOASA-N |
SMILES |
C1=CC=C(C=C1)COCC2C(C(C(O2)O)OCC3=CC=CC=C3)OCC4=CC=CC=C4 |
異性体SMILES |
C1=CC=C(C=C1)COC[C@H]2[C@@H](C(C(O2)O)OCC3=CC=CC=C3)OCC4=CC=CC=C4 |
正規SMILES |
C1=CC=C(C=C1)COCC2C(C(C(O2)O)OCC3=CC=CC=C3)OCC4=CC=CC=C4 |
製品の起源 |
United States |
Retrosynthesis Analysis
AI-Powered Synthesis Planning: Our tool employs the Template_relevance Pistachio, Template_relevance Bkms_metabolic, Template_relevance Pistachio_ringbreaker, Template_relevance Reaxys, Template_relevance Reaxys_biocatalysis model, leveraging a vast database of chemical reactions to predict feasible synthetic routes.
One-Step Synthesis Focus: Specifically designed for one-step synthesis, it provides concise and direct routes for your target compounds, streamlining the synthesis process.
Accurate Predictions: Utilizing the extensive PISTACHIO, BKMS_METABOLIC, PISTACHIO_RINGBREAKER, REAXYS, REAXYS_BIOCATALYSIS database, our tool offers high-accuracy predictions, reflecting the latest in chemical research and data.
Strategy Settings
Precursor scoring | Relevance Heuristic |
---|---|
Min. plausibility | 0.01 |
Model | Template_relevance |
Template Set | Pistachio/Bkms_metabolic/Pistachio_ringbreaker/Reaxys/Reaxys_biocatalysis |
Top-N result to add to graph | 6 |
Feasible Synthetic Routes
Q1: What is the primary use of 2,3,5-Tri-O-benzyl-D-ribofuranose in organic synthesis?
A1: This compound serves as a versatile precursor in the synthesis of C-nucleosides [, , , , , ] and various ribofuranosides [, , , , , ]. These molecules are of significant interest due to their potential antiviral and anticancer activities.
Q2: Can you elaborate on the synthesis of β-D-ribofuranosides using this compound?
A2: Several methods have been developed for the stereoselective synthesis of β-D-ribofuranosides from 2,3,5-tri-O-benzyl-D-ribofuranose:
- [Catecholato(2−)-O,O′]oxotitanium/Trifluoromethanesulfonic Anhydride: This approach utilizes the combination of these reagents and trimethylsilylated nucleophiles to provide β-D-ribofuranosides in high yields [].
- Silver Salts and Lawesson’s Reagent or Diphenyltin Sulfide: This catalytic system, employing either Lawesson’s reagent ([2,4-bis(4-methoxyphenyl)-1,3-dithia-2,4-diphosphetane-2,4-disulfide]) or diphenyltin sulfide (Ph2Sn=S), facilitates the efficient coupling of 2,3,5-tri-O-benzyl-D-ribofuranose with alcohols to produce β-D-ribofuranosides under mild conditions [, ].
- Trityl Salt Catalysis: In the presence of catalytic amounts of trityl salts, 2,3,5-tri-O-benzyl-D-ribofuranose reacts smoothly with alcohols to afford β-ribofuranosides in high yields with excellent stereoselectivity [].
Q3: What about the synthesis of α-D-ribofuranosides? Is stereoselective synthesis possible?
A3: Yes, achieving α-D-ribofuranoside selectivity is possible through different strategies:
- Lithium Perchlorate as an Additive: The addition of lithium perchlorate during glycosylation reactions, especially when using silver salts as catalysts and 2,3,5-tri-O-benzyl-1-O-iodoacetyl-D-ribofuranose as a starting material, promotes the formation of α-D-ribofuranosides in high yields [].
- Lithium Bis[(trifluoromethyl)sulfonyl]imide Influence: This lithium salt exhibits a significant effect on the stereochemical outcome, favoring the formation of α-D-ribofuranosides when reacting 2,3,5-tri-O-benzyl-D-ribofuranose with alcohols [].
Q4: Can 2,3,5-tri-O-benzyl-D-ribofuranose be used to synthesize C-nucleosides other than pyrazole derivatives?
A4: Absolutely. Research demonstrates its successful utilization in synthesizing C-nucleosides incorporating various aromatic heterocycles as base moieties []. For instance, coupling it with magnesium, cadmium, or zinc salts of these heterocycles leads to the stereoselective formation of the corresponding β-C-nucleosides.
Q5: Has 2,3,5-tri-O-benzyl-D-ribofuranose been used in the synthesis of any other interesting carbohydrate derivatives?
A5: Yes, besides C-nucleosides and ribofuranosides, this compound has been employed in the synthesis of chiral dienones. For example, aldol reaction of 2,3,5-tri-O-benzyl-D-ribofuranose with acetone under basic conditions provides a (3E,5Z,7S)-octa-3,5-dien-2-one derivative [].
試験管内研究製品の免責事項と情報
BenchChemで提示されるすべての記事および製品情報は、情報提供を目的としています。BenchChemで購入可能な製品は、生体外研究のために特別に設計されています。生体外研究は、ラテン語の "in glass" に由来し、生物体の外で行われる実験を指します。これらの製品は医薬品または薬として分類されておらず、FDAから任何の医療状態、病気、または疾患の予防、治療、または治癒のために承認されていません。これらの製品を人間または動物に体内に導入する形態は、法律により厳格に禁止されています。これらのガイドラインに従うことは、研究と実験において法的および倫理的な基準の遵守を確実にするために重要です。