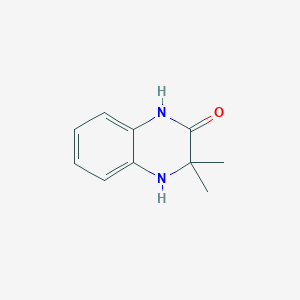
3,3-dimethyl-3,4-dihydroquinoxalin-2(1H)-one
概要
説明
3,3-Dimethyl-3,4-dihydroquinoxalin-2(1H)-one is a heterocyclic organic compound with the molecular formula C10H12N2O. It belongs to the quinoxaline family, which is known for its diverse biological activities and applications in medicinal chemistry. This compound features a quinoxaline core with a 3,3-dimethyl substitution and a 3,4-dihydro configuration, making it an interesting subject for chemical and pharmaceutical research.
準備方法
Synthetic Routes and Reaction Conditions
The synthesis of 3,3-dimethyl-3,4-dihydroquinoxalin-2(1H)-one typically involves the cyclization of appropriate precursors under controlled conditions. One common method includes the condensation of 3,3-dimethyl-1,2-diaminopropane with a suitable diketone, such as glyoxal, under acidic or basic conditions. The reaction is usually carried out in a solvent like ethanol or methanol, and the product is purified through recrystallization.
Industrial Production Methods
On an industrial scale, the production of this compound may involve continuous flow processes to ensure high yield and purity. The use of automated reactors and precise control of reaction parameters, such as temperature, pressure, and pH, can optimize the synthesis. Additionally, advanced purification techniques like chromatography and crystallization are employed to obtain the compound in its pure form.
化学反応の分析
Types of Reactions
3,3-Dimethyl-3,4-dihydroquinoxalin-2(1H)-one undergoes various chemical reactions, including:
Oxidation: The compound can be oxidized to form quinoxaline derivatives.
Reduction: Reduction reactions can convert it into more saturated analogs.
Substitution: Nucleophilic and electrophilic substitution reactions can introduce different functional groups onto the quinoxaline core.
Common Reagents and Conditions
Oxidation: Common oxidizing agents include potassium permanganate (KMnO4) and hydrogen peroxide (H2O2).
Reduction: Reducing agents like sodium borohydride (NaBH4) and lithium aluminum hydride (LiAlH4) are often used.
Substitution: Reagents such as halogens (e.g., bromine, chlorine) and organometallic compounds (e.g., Grignard reagents) facilitate substitution reactions.
Major Products
The major products formed from these reactions depend on the specific conditions and reagents used. For example, oxidation may yield quinoxaline-2,3-dione, while reduction can produce 3,3-dimethyl-3,4-dihydroquinoxaline. Substitution reactions can introduce various functional groups, leading to a wide range of derivatives with potential biological activities.
科学的研究の応用
Medicinal Chemistry
1.1 Antimicrobial Activity
Research indicates that quinoxaline derivatives, including 3,3-dimethyl-3,4-dihydroquinoxalin-2(1H)-one, exhibit significant antimicrobial properties. These compounds have been synthesized and tested against various bacterial strains, demonstrating effectiveness comparable to established antibiotics. A study highlighted the compound's ability to inhibit the growth of both Gram-positive and Gram-negative bacteria, suggesting potential as a lead compound for developing new antimicrobial agents .
1.2 Anticancer Properties
The compound has also shown promise in cancer research. A series of derivatives were synthesized and evaluated for their cytotoxic effects on cancer cell lines. The results indicated that modifications to the quinoxaline structure could enhance antiproliferative activity against specific cancer types, making it a candidate for further development in cancer therapeutics .
1.3 Neuroprotective Effects
Emerging studies suggest that this compound may possess neuroprotective properties. It has been investigated for its ability to protect neuronal cells from oxidative stress and apoptosis. This characteristic could be beneficial in treating neurodegenerative diseases such as Alzheimer's and Parkinson's disease .
Material Science Applications
2.1 Photoluminescent Materials
The unique electronic properties of quinoxaline derivatives have led to their exploration as photoluminescent materials. Research has demonstrated that incorporating this compound into polymer matrices can enhance the luminescent properties of the resulting materials, making them suitable for applications in organic light-emitting diodes (OLEDs) and other optoelectronic devices .
2.2 Organic Synthesis
In organic synthesis, this compound serves as an important intermediate for producing more complex molecules. Its reactivity allows it to participate in various chemical reactions, including cycloadditions and couplings, facilitating the synthesis of diverse organic compounds with potential applications in pharmaceuticals and agrochemicals .
Case Study 1: Antibacterial Activity Evaluation
In a controlled study published in the Journal of Medicinal Chemistry, researchers synthesized various derivatives of this compound and evaluated their antibacterial activity against resistant strains of Staphylococcus aureus. The study found that certain modifications significantly enhanced antibacterial efficacy compared to standard treatments .
Case Study 2: Neuroprotective Mechanism Investigation
A recent investigation published in Neuropharmacology examined the neuroprotective effects of this compound on cultured neuronal cells exposed to oxidative stressors. The findings revealed that treatment with this compound reduced cell death by inhibiting apoptotic pathways and enhancing antioxidant defenses .
作用機序
The mechanism of action of 3,3-dimethyl-3,4-dihydroquinoxalin-2(1H)-one involves its interaction with molecular targets such as enzymes and receptors. The compound can inhibit or activate these targets, leading to various biological effects. For instance, it may inhibit bacterial enzymes, thereby exhibiting antimicrobial activity. The exact pathways and molecular interactions depend on
生物活性
3,3-Dimethyl-3,4-dihydroquinoxalin-2(1H)-one is a compound of interest due to its potential biological activities, particularly in the fields of neurodegenerative diseases and cancer treatment. This article reviews the biological activity of this compound, focusing on its mechanisms of action, therapeutic potentials, and relevant case studies.
This compound belongs to the quinoxaline family, which has been extensively studied for various pharmacological properties. The structural formula can be represented as follows:
The biological activities of this compound are primarily attributed to its interaction with various enzymes and receptors:
- Inhibition of Acetylcholinesterase (AChE) : Studies have shown that derivatives of quinoxaline can inhibit AChE, an enzyme involved in the breakdown of acetylcholine, thereby enhancing cholinergic transmission. This is particularly relevant for conditions like Alzheimer's disease .
- Monoamine Oxidase (MAO) Inhibition : The compound has demonstrated potential as a dual inhibitor of MAO-A and MAO-B, which play significant roles in neurodegenerative diseases. It has been reported that certain derivatives exhibit IC50 values in the low micromolar range against these enzymes .
Anticancer Activity
Research indicates that quinoxaline derivatives exhibit significant anticancer properties. In a study involving various cancer cell lines:
Compound | Cell Line | IC50 (µM) |
---|---|---|
3e | Ty-82 | 2.5 |
3e | THP-1 | 1.6 |
49 | HCT116 | 0.0029 |
49 | MCF-7 | 0.071 |
Compound 49 showed exceptional potency against MAO-B with an IC50 value of 0.0029 µM . These findings underscore the potential of this compound as a lead compound for developing anticancer agents.
Neuroprotective Effects
The compound's ability to cross the blood-brain barrier (BBB) enhances its therapeutic potential for neurodegenerative diseases. In vitro studies demonstrated that it does not exhibit cytotoxicity at concentrations lower than 12.5 µM in neuronal cell lines . This suggests a favorable safety profile for potential therapeutic use.
Case Studies
- Alzheimer’s Disease : A study synthesized various derivatives based on the core structure of 3,4-dihydroquinoxalin-2(1H)-one and evaluated their inhibitory effects on AChE and MAOs. The most promising candidate showed balanced inhibition and favorable pharmacokinetics .
- Cancer Treatment : In another investigation involving multiple cancer cell lines, compounds derived from quinoxaline were tested for growth inhibition. Notably, one derivative achieved over 55% growth inhibition against melanoma cells .
特性
IUPAC Name |
3,3-dimethyl-1,4-dihydroquinoxalin-2-one | |
---|---|---|
Source | PubChem | |
URL | https://pubchem.ncbi.nlm.nih.gov | |
Description | Data deposited in or computed by PubChem | |
InChI |
InChI=1S/C10H12N2O/c1-10(2)9(13)11-7-5-3-4-6-8(7)12-10/h3-6,12H,1-2H3,(H,11,13) | |
Source | PubChem | |
URL | https://pubchem.ncbi.nlm.nih.gov | |
Description | Data deposited in or computed by PubChem | |
InChI Key |
RDKNTOVJVFYGPA-UHFFFAOYSA-N | |
Source | PubChem | |
URL | https://pubchem.ncbi.nlm.nih.gov | |
Description | Data deposited in or computed by PubChem | |
Canonical SMILES |
CC1(C(=O)NC2=CC=CC=C2N1)C | |
Source | PubChem | |
URL | https://pubchem.ncbi.nlm.nih.gov | |
Description | Data deposited in or computed by PubChem | |
Molecular Formula |
C10H12N2O | |
Source | PubChem | |
URL | https://pubchem.ncbi.nlm.nih.gov | |
Description | Data deposited in or computed by PubChem | |
DSSTOX Substance ID |
DTXSID70344226 | |
Record name | 3,3-dimethyl-3,4-dihydroquinoxalin-2(1H)-one | |
Source | EPA DSSTox | |
URL | https://comptox.epa.gov/dashboard/DTXSID70344226 | |
Description | DSSTox provides a high quality public chemistry resource for supporting improved predictive toxicology. | |
Molecular Weight |
176.21 g/mol | |
Source | PubChem | |
URL | https://pubchem.ncbi.nlm.nih.gov | |
Description | Data deposited in or computed by PubChem | |
CAS No. |
80636-30-2 | |
Record name | 3,3-dimethyl-3,4-dihydroquinoxalin-2(1H)-one | |
Source | EPA DSSTox | |
URL | https://comptox.epa.gov/dashboard/DTXSID70344226 | |
Description | DSSTox provides a high quality public chemistry resource for supporting improved predictive toxicology. | |
Record name | 3,3-dimethyl-1,2,3,4-tetrahydroquinoxalin-2-one | |
Source | European Chemicals Agency (ECHA) | |
URL | https://echa.europa.eu/information-on-chemicals | |
Description | The European Chemicals Agency (ECHA) is an agency of the European Union which is the driving force among regulatory authorities in implementing the EU's groundbreaking chemicals legislation for the benefit of human health and the environment as well as for innovation and competitiveness. | |
Explanation | Use of the information, documents and data from the ECHA website is subject to the terms and conditions of this Legal Notice, and subject to other binding limitations provided for under applicable law, the information, documents and data made available on the ECHA website may be reproduced, distributed and/or used, totally or in part, for non-commercial purposes provided that ECHA is acknowledged as the source: "Source: European Chemicals Agency, http://echa.europa.eu/". Such acknowledgement must be included in each copy of the material. ECHA permits and encourages organisations and individuals to create links to the ECHA website under the following cumulative conditions: Links can only be made to webpages that provide a link to the Legal Notice page. | |
Synthesis routes and methods I
Procedure details
Synthesis routes and methods II
Procedure details
Synthesis routes and methods III
Procedure details
Q1: What are the key steps involved in the synthesis of 7-Bromo-8-methoxycarbonyl-3,3-dimethyl-3,4-dihydro-1H-quinoxalin-2-one?
A1: While the provided abstracts do not detail the complete synthetic route, one paper focuses on developing a practical synthesis for 7-Bromo-8-methoxycarbonyl-3,3-dimethyl-3,4-dihydro-1H-quinoxalin-2-one []. This suggests that the synthesis likely involves multiple steps and that researchers are actively seeking optimized and efficient methods for its production. The presence of bromine and methoxycarbonyl substituents on the core structure indicates further modifications targeting specific properties or applications. To gain a comprehensive understanding of the synthesis, referring to the full research article [] would be necessary.
Q2: Why is there interest in developing a process for producing 3,3-Dimethyl-3,4-dihydro-1H-quinoxalin-2-one?
A2: One of the provided research articles focuses on developing a process for producing 3,3-Dimethyl-3,4-dihydro-1H-quinoxalin-2-one []. This suggests that this compound is likely an important intermediate in the synthesis of other, potentially more complex and valuable molecules. The development of an efficient production process for this compound is crucial for facilitating the synthesis of these downstream products. Further research exploring the applications of these downstream products would provide a more complete understanding of the significance of 3,3-Dimethyl-3,4-dihydro-1H-quinoxalin-2-one.
試験管内研究製品の免責事項と情報
BenchChemで提示されるすべての記事および製品情報は、情報提供を目的としています。BenchChemで購入可能な製品は、生体外研究のために特別に設計されています。生体外研究は、ラテン語の "in glass" に由来し、生物体の外で行われる実験を指します。これらの製品は医薬品または薬として分類されておらず、FDAから任何の医療状態、病気、または疾患の予防、治療、または治癒のために承認されていません。これらの製品を人間または動物に体内に導入する形態は、法律により厳格に禁止されています。これらのガイドラインに従うことは、研究と実験において法的および倫理的な基準の遵守を確実にするために重要です。