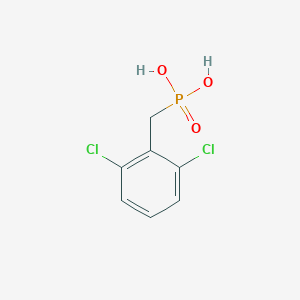
2,6-Dichlorobenzylphosphonic acid
概要
説明
2,6-Dichlorobenzylphosphonic acid is an organophosphorus compound with the molecular formula C7H7Cl2O3P It features a benzene ring substituted with two chlorine atoms at the 2 and 6 positions and a phosphonic acid group
科学的研究の応用
2,6-Dichlorobenzylphosphonic acid has several scientific research applications:
Chemistry: It is used as a reagent in organic synthesis and as a precursor for other organophosphorus compounds.
Biology: The compound is studied for its potential biological activity, including its effects on enzymes and cellular processes.
Medicine: Research is ongoing into its potential use as a therapeutic agent, particularly in the development of enzyme inhibitors.
Industry: It is used in the production of flame retardants, plasticizers, and other industrial chemicals
Safety and Hazards
将来の方向性
準備方法
Synthetic Routes and Reaction Conditions
2,6-Dichlorobenzylphosphonic acid can be synthesized through several methods. One common approach involves the reaction of 2,6-dichlorobenzyl chloride with a phosphite ester, followed by hydrolysis to yield the phosphonic acid. The reaction typically requires a base such as sodium hydroxide and is conducted under reflux conditions .
Industrial Production Methods
Industrial production of this compound often involves the dealkylation of dialkyl phosphonates under acidic conditions (e.g., using hydrochloric acid) or via the McKenna procedure, which uses bromotrimethylsilane followed by methanolysis .
化学反応の分析
Types of Reactions
2,6-Dichlorobenzylphosphonic acid undergoes various chemical reactions, including:
Substitution Reactions: The chlorine atoms can be substituted by nucleophiles under appropriate conditions.
Oxidation and Reduction: The phosphonic acid group can participate in redox reactions, although these are less common.
Hydrolysis: The ester forms of this compound can be hydrolyzed to yield the free phosphonic acid.
Common Reagents and Conditions
Common reagents used in reactions with this compound include bases (e.g., sodium hydroxide), acids (e.g., hydrochloric acid), and nucleophiles (e.g., amines). Reaction conditions often involve refluxing in solvents such as ethanol or water .
Major Products
The major products formed from these reactions depend on the specific reagents and conditions used. For example, substitution reactions can yield various substituted benzylphosphonic acids, while hydrolysis yields the free phosphonic acid.
作用機序
The mechanism of action of 2,6-dichlorobenzylphosphonic acid involves its interaction with molecular targets such as enzymes. The phosphonic acid group can mimic phosphate groups, allowing the compound to inhibit enzymes that utilize phosphate substrates. This inhibition can affect various biochemical pathways, making the compound useful in research and potential therapeutic applications .
類似化合物との比較
Similar Compounds
Benzylphosphonic acid: Lacks the chlorine substituents, making it less reactive in certain substitution reactions.
2,4-Dichlorobenzylphosphonic acid: Similar structure but with chlorine atoms at different positions, affecting its reactivity and biological activity.
Phenylphosphonic acid: Lacks the benzyl group, resulting in different chemical properties and applications.
Uniqueness
2,6-Dichlorobenzylphosphonic acid is unique due to the presence of chlorine atoms at the 2 and 6 positions, which influence its reactivity and potential applications. The specific positioning of these substituents can enhance its effectiveness as an enzyme inhibitor and its utility in various chemical reactions.
特性
IUPAC Name |
(2,6-dichlorophenyl)methylphosphonic acid | |
---|---|---|
Source | PubChem | |
URL | https://pubchem.ncbi.nlm.nih.gov | |
Description | Data deposited in or computed by PubChem | |
InChI |
InChI=1S/C7H7Cl2O3P/c8-6-2-1-3-7(9)5(6)4-13(10,11)12/h1-3H,4H2,(H2,10,11,12) | |
Source | PubChem | |
URL | https://pubchem.ncbi.nlm.nih.gov | |
Description | Data deposited in or computed by PubChem | |
InChI Key |
CPPHQOFUDIOVLN-UHFFFAOYSA-N | |
Source | PubChem | |
URL | https://pubchem.ncbi.nlm.nih.gov | |
Description | Data deposited in or computed by PubChem | |
Canonical SMILES |
C1=CC(=C(C(=C1)Cl)CP(=O)(O)O)Cl | |
Source | PubChem | |
URL | https://pubchem.ncbi.nlm.nih.gov | |
Description | Data deposited in or computed by PubChem | |
Molecular Formula |
C7H7Cl2O3P | |
Source | PubChem | |
URL | https://pubchem.ncbi.nlm.nih.gov | |
Description | Data deposited in or computed by PubChem | |
DSSTOX Substance ID |
DTXSID00365282 | |
Record name | 2,6-dichlorobenzylphosphonic acid | |
Source | EPA DSSTox | |
URL | https://comptox.epa.gov/dashboard/DTXSID00365282 | |
Description | DSSTox provides a high quality public chemistry resource for supporting improved predictive toxicology. | |
Molecular Weight |
241.00 g/mol | |
Source | PubChem | |
URL | https://pubchem.ncbi.nlm.nih.gov | |
Description | Data deposited in or computed by PubChem | |
CAS No. |
80395-10-4 | |
Record name | 2,6-dichlorobenzylphosphonic acid | |
Source | EPA DSSTox | |
URL | https://comptox.epa.gov/dashboard/DTXSID00365282 | |
Description | DSSTox provides a high quality public chemistry resource for supporting improved predictive toxicology. | |
Synthesis routes and methods
Procedure details
Retrosynthesis Analysis
AI-Powered Synthesis Planning: Our tool employs the Template_relevance Pistachio, Template_relevance Bkms_metabolic, Template_relevance Pistachio_ringbreaker, Template_relevance Reaxys, Template_relevance Reaxys_biocatalysis model, leveraging a vast database of chemical reactions to predict feasible synthetic routes.
One-Step Synthesis Focus: Specifically designed for one-step synthesis, it provides concise and direct routes for your target compounds, streamlining the synthesis process.
Accurate Predictions: Utilizing the extensive PISTACHIO, BKMS_METABOLIC, PISTACHIO_RINGBREAKER, REAXYS, REAXYS_BIOCATALYSIS database, our tool offers high-accuracy predictions, reflecting the latest in chemical research and data.
Strategy Settings
Precursor scoring | Relevance Heuristic |
---|---|
Min. plausibility | 0.01 |
Model | Template_relevance |
Template Set | Pistachio/Bkms_metabolic/Pistachio_ringbreaker/Reaxys/Reaxys_biocatalysis |
Top-N result to add to graph | 6 |
Feasible Synthetic Routes
試験管内研究製品の免責事項と情報
BenchChemで提示されるすべての記事および製品情報は、情報提供を目的としています。BenchChemで購入可能な製品は、生体外研究のために特別に設計されています。生体外研究は、ラテン語の "in glass" に由来し、生物体の外で行われる実験を指します。これらの製品は医薬品または薬として分類されておらず、FDAから任何の医療状態、病気、または疾患の予防、治療、または治癒のために承認されていません。これらの製品を人間または動物に体内に導入する形態は、法律により厳格に禁止されています。これらのガイドラインに従うことは、研究と実験において法的および倫理的な基準の遵守を確実にするために重要です。