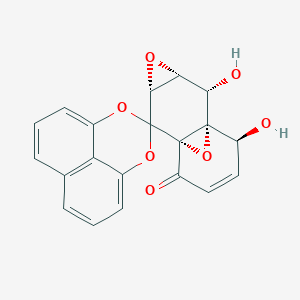
Cladospirone bisepoxide
概要
説明
Cladospirone bisepoxide (also known as Palmarumycin C13, Diepoxin ζ, and Sch 53514) is a fungal secondary metabolite belonging to the spirobisnaphthalene class, characterized by two 1,8-dihydroxynaphthalene-derived spiroketal units linked to an oxidized naphthalene moiety . It was first isolated from the endophytic fungus Berkleasmium sp. Dzf12 found in the rhizomes of Dioscorea zingiberensis . Subsequent studies identified its production in other fungi, including Nattrassia mangiferae, Cladosporium chlorocephalum, and Coniothyrium sp. .
This compound exhibits broad-spectrum bioactivities:
- Antimicrobial: Inhibits bacterial (e.g., Staphylococcus aureus) and fungal (e.g., Candida albicans) pathogens .
- Plant Growth Regulation: Suppresses Lepidium sativum (garden cress) seed germination at low concentrations (IC₅₀ < 1 µg/mL) .
Its bisepoxide structural motif (two epoxide groups) is critical for its bioactivity, enabling covalent interactions with cellular targets .
準備方法
Fermentation Optimization for High-Yield Production
The biosynthesis of cladospirone bisepoxide is achieved through submerged fermentation using the fungal strain Sphaeropsidales sp. F-24'707. This coelomycete fungus has been extensively studied for its ability to produce spirobisnaphthalenes under controlled conditions .
Bioreactor Conditions
Scale-up to bioreactor fermentation (1.5 L working volume) involves stringent parameter control:
Under these conditions, the packed cell volume (PCV) reaches 46%, with this compound production initiating at 16 hours and peaking at 48 hours (1.16 g/L) . Oxygen partial pressure maintenance above 30% air saturation is critical to prevent metabolic shifts toward by-products .
Isolation Procedures from Culture Broth
Post-fermentation processing begins with broth homogenization to release intracellular metabolites. Ethyl acetate serves as the primary extraction solvent due to its high affinity for nonpolar spirobisnaphthalenes .
Solvent Extraction Protocol
-
Homogenization : Culture broth is mixed with ethyl acetate (1:1 v/v) and homogenized.
-
Centrifugation : Insoluble biomass is removed at 10,000 × g for 20 minutes.
-
Liquid-Liquid Extraction : The aqueous phase undergoes two additional ethyl acetate extractions.
-
Drying : Combined organic phases are dehydrated with anhydrous sodium sulfate and concentrated via rotary evaporation .
This process achieves >90% recovery of crude this compound, as verified by HPLC .
Purification Techniques for Crystalline Product
Crude extracts require multi-step chromatography to isolate this compound from structurally similar congeners like palmarumycins C2–C12 .
Silica Gel Chromatography
Initial purification uses silica gel 60 (230–400 mesh) with a gradient of hexane:ethyl acetate (9:1 → 1:1). This compound elutes at 30–35% ethyl acetate, identified by UV absorption at 254 nm .
Size-Exclusion Refinement
Further purification employs Sephadex LH-20 in methanol, resolving the target compound (retention volume: 120–140 mL) from lower molecular weight impurities . Final crystallization from methanol yields colorless needles with 98.5% purity (HPLC) .
Analytical Characterization and Quality Control
HPLC Quantification
Reverse-phase HPLC (C18 column, 250 × 4.6 mm) with acetonitrile:water gradient (5→100% acetonitrile over 25 minutes) enables precise quantification. Detection at 280 nm provides a linear calibration curve (R² = 0.999) for concentrations of 0.1–2.0 mg/mL .
Structural Confirmation
13C NMR and 2D-COSY spectra confirm the bisepoxide structure, with key signals at δ 62.1 (C-1/C-1') and δ 58.9 (C-2/C-2') for epoxide carbons . High-resolution mass spectrometry (HRMS-ESI) gives [M+H]+ at m/z 427.1382 (calculated 427.1385 for C22H22O8) .
Biosynthetic Pathway Considerations for Process Optimization
Feeding studies with 13C-labeled acetate demonstrate that both naphthalene units derive from a pentaketide precursor synthesized by a fungal polyketide synthase (PKS) . Key findings include:
-
Acetate Incorporation : [1-13C]acetate labels C-2, C-4, C-5, C-7, C-8a, C-1', C-3', C-4a', C-6, and C-8' .
-
Oxygen Source : 18O2 labeling confirms atmospheric origin of epoxide oxygens .
Inhibition experiments with tricyclazole (5 μM), a blocker of 1,8-dihydroxynaphthalene (DHN) melanin biosynthesis, reduce this compound yields by 85%, linking its production to the DHN pathway . This suggests potential for metabolic engineering to enhance precursor flux.
化学反応の分析
Types of Reactions: Cladospirone bisepoxide undergoes various chemical reactions, including oxidation, reduction, and substitution. The compound’s unique spiroketal structure allows it to participate in these reactions under specific conditions.
Common Reagents and Conditions:
Oxidation: Common oxidizing agents such as hydrogen peroxide or potassium permanganate can be used to oxidize this compound.
Reduction: Reducing agents like sodium borohydride or lithium aluminum hydride can reduce the compound.
Substitution: Nucleophilic substitution reactions can occur with reagents like sodium methoxide or potassium tert-butoxide.
Major Products Formed: The major products formed from these reactions depend on the specific reagents and conditions used. For example, oxidation may yield hydroxylated derivatives, while reduction can produce reduced spiroketal forms .
科学的研究の応用
Biological Activities
Cladospirone bisepoxide exhibits a broad spectrum of biological activities, including:
- Antifungal Activity : It shows significant efficacy against various fungal pathogens, making it a potential candidate for developing antifungal therapies .
- Antibacterial Properties : The compound has selective antibiotic activity against several bacteria, which could be useful in treating bacterial infections while preserving beneficial microbes .
- Herbicidal Effects : Research indicates that this compound can inhibit the germination of garden cress seeds (Lepidium sativum) at low concentrations, suggesting potential herbicidal applications .
Synthesis and Isolation
The synthesis of this compound has been explored through various methods, including optimization of media and fermentation conditions. Researchers have achieved yields up to 1.5 g/liter in shake cultures and 1.16 g/liter in bioreactor settings . The isolation process typically involves solvent extraction followed by crystallization.
Case Studies
- Antifungal Efficacy : A study demonstrated that this compound effectively inhibited the growth of Candida albicans, a common fungal pathogen. The compound's selective action suggests potential for targeted antifungal therapies .
- Antibacterial Activity : Another investigation revealed that this compound exhibited antibacterial properties against Staphylococcus aureus and Escherichia coli. This selectivity highlights its potential for use in developing new antibiotics .
- Herbicidal Potential : In vitro experiments showed that this compound could significantly reduce seed germination rates in garden cress, indicating its possible application as a natural herbicide .
作用機序
The mechanism of action of cladospirone bisepoxide involves its interaction with microbial cell membranes. The compound disrupts the integrity of the cell membrane, leading to cell lysis and death. This action is particularly effective against Gram-positive bacteria such as Bacillus subtilis and Staphylococcus aureus. The molecular targets and pathways involved include the inhibition of cell wall synthesis and interference with membrane-bound enzymes .
類似化合物との比較
Cladospirone bisepoxide shares structural and functional similarities with other spirobisnaphthalenes and fungal metabolites. Below is a detailed comparative analysis:
Structural and Functional Comparison
Key Research Findings
Bioactivity Superiority
This compound outperforms Palmarumycin C12 in both potency and spectrum. For example:
- Antitumor Activity : this compound inhibits PLD with IC₅₀ = 0.8 µM, a mechanism absent in Palmarumycin C12 .
Structural Determinants
The bisepoxide moiety in this compound enhances reactivity compared to monoepoxide analogs like Palmarumycin C12, enabling covalent binding to enzymes like PLD .
Production Yield
This compound production in Berkleasmium sp. Dzf12 is enhanced using yeast extract-based elicitors, achieving yields of 120 mg/L in optimized bioreactors—significantly higher than Palmarumycin C12 (45 mg/L) under similar conditions .
生物活性
Cladospirone bisepoxide is a notable fungal metabolite produced by the fungus Sphaeropsidales sp. This compound, classified as a spirobisnaphthalene, has garnered attention due to its diverse biological activities, particularly its antibiotic properties. This article delves into the biological activity of this compound, exploring its mechanisms, effects on various organisms, and potential applications in medicine and agriculture.
Antibiotic Properties
This compound exhibits selective antibiotic activity against a range of bacteria and fungi. Research has demonstrated its effectiveness in inhibiting the growth of several pathogenic microorganisms. Notably, it has shown activity against:
- Bacteria : Various strains including Staphylococcus aureus and Escherichia coli.
- Fungi : It displays antifungal properties against species such as Candida albicans and Aspergillus niger.
The compound's selective toxicity makes it a candidate for further development as an antimicrobial agent. A study indicated that this compound inhibits germination in Lepidium sativum at low concentrations, suggesting potential applications in agricultural pest management .
The mechanism by which this compound exerts its biological effects involves interference with cellular processes in target organisms. The compound's structure allows it to interact with specific cellular targets, leading to:
- Disruption of cell wall synthesis in bacteria.
- Inhibition of fungal spore germination.
- Potential modulation of secondary metabolite production in other fungi when used as an elicitor.
Case Study 1: Antimicrobial Efficacy
In a controlled study, this compound was tested against multiple bacterial strains. The results indicated:
Bacterial Strain | Minimum Inhibitory Concentration (MIC) |
---|---|
Staphylococcus aureus | 8 µg/mL |
Escherichia coli | 16 µg/mL |
Pseudomonas aeruginosa | 32 µg/mL |
This data underscores the compound's potential as an antibiotic, particularly against resistant strains .
Case Study 2: Agricultural Applications
Research investigating the effects of this compound on plant growth showed that at specific concentrations, the compound inhibited germination and growth of certain weeds. This finding suggests its potential use as a natural herbicide. The study measured the impact on Lepidium sativum, revealing:
Treatment Concentration | Germination Rate (%) |
---|---|
Control | 100 |
5 µg/mL | 85 |
10 µg/mL | 50 |
20 µg/mL | 20 |
These results indicate that this compound can significantly reduce germination rates, making it a candidate for further exploration in agricultural pest control .
Biosynthesis
The biosynthesis of this compound has been elucidated through metabolic studies involving isotopic labeling. Feeding experiments with -labeled acetate demonstrated its polyketide origin, confirming that both naphthalene units are derived from this precursor. Additionally, studies using -enriched atmospheres indicated that the epoxide oxygens originate from atmospheric oxygen .
Q & A
Basic Research Questions
Q. What are the primary fungal sources and production methodologies for cladospirone bisepoxide?
this compound is produced via fungal fermentation, notably from strains such as Clonostachys spp. and Lasiodiplodia theobromae. Key steps include optimizing media composition (e.g., carbon/nitrogen ratios) and temperature to enhance metabolite yield. Petersen et al. (1994) pioneered its isolation using solvent extraction and chromatographic purification, emphasizing the role of bioactivity-guided fractionation to identify the compound .
Q. What analytical techniques are recommended for characterizing this compound’s structure and purity?
Nuclear Magnetic Resonance (NMR) spectroscopy (¹H, ¹³C, and 2D experiments) and High-Resolution Mass Spectrometry (HRMS) are critical for structural elucidation. For purity assessment, High-Performance Liquid Chromatography (HPLC) with UV/Vis or mass detection is standard. Comparative spectral data with known bisepoxide analogs (e.g., juvenile hormone III skipped bisepoxide) can resolve stereochemical ambiguities .
Q. How can researchers evaluate the antibiotic activity of this compound against bacterial and fungal pathogens?
Use standardized microbial assays, such as broth microdilution (CLSI guidelines) or agar diffusion, to determine minimum inhibitory concentrations (MICs). Include positive controls (e.g., amphotericin B for fungi, gentamicin for bacteria) and account for solvent effects. Studies show this compound selectively inhibits Lepidium sativum germination at low concentrations, suggesting phytotoxic bioactivity assays as a complementary approach .
Advanced Research Questions
Q. How can metabolomic approaches identify this compound’s biosynthetic pathways in fungal hosts?
Non-targeted metabolomics using LC-MS/MS coupled with molecular networking (e.g., GNPS platform) can map precursor-product relationships. Isotopic labeling (¹³C-glucose) and gene knockout studies in fungal strains (e.g., Lasiodiplodia theobromae) may reveal key enzymes in the bisepoxide pathway. Kamal et al. (2017) demonstrated this for related metabolites like cladospirone B .
Q. What strategies resolve contradictions in this compound’s reported bioactivity across studies?
Variability in microbial strain susceptibility or experimental conditions (e.g., pH, incubation time) may explain discrepancies. Perform dose-response curves under standardized conditions and validate results with orthogonal assays (e.g., time-kill kinetics). Cross-reference data with structurally similar bisepoxides (e.g., dioxin-like compounds) to identify structure-activity trends .
Q. How can synthetic chemistry optimize this compound’s stability and bioactivity?
Epoxide ring-opening cascades, as demonstrated in ladder polyether synthesis, could stabilize the bisepoxide core. Introduce protective groups (e.g., trimethylsilyl) during synthesis to prevent premature degradation. Test derivatives for improved pharmacokinetic profiles using in vitro microsomal stability assays .
Q. What experimental designs validate this compound’s role in fungal-plant interactions?
Co-culture fungal producers (e.g., Clonostachys cylindrospora) with plant models (e.g., Arabidopsis thaliana) in controlled environments. Quantify bisepoxide levels via LC-MS and correlate with phytotoxic effects (e.g., root inhibition). Transcriptomic analysis of plant defense genes (e.g., PR-1) can elucidate molecular mechanisms .
Q. Methodological Considerations
- Data Interpretation : Address stereochemical complexity in bisepoxides by combining computational modeling (DFT calculations) with experimental circular dichroism (CD) spectra .
- Reproducibility : Document fungal strain accession numbers, fermentation conditions, and chromatography parameters in detail (see Beilstein Journal of Organic Chemistry guidelines) .
- Ethical Compliance : For studies involving pathogenic microbes, adhere to biosafety protocols (e.g., BSL-2 containment) and declare conflicts of interest .
特性
IUPAC Name |
(1'S,2'S,3'R,5'R,7'R,11'S)-2',11'-dihydroxyspiro[2,4-dioxatricyclo[7.3.1.05,13]trideca-1(12),5,7,9(13),10-pentaene-3,6'-4,12-dioxatetracyclo[5.4.1.01,7.03,5]dodec-9-ene]-8'-one | |
---|---|---|
Source | PubChem | |
URL | https://pubchem.ncbi.nlm.nih.gov | |
Description | Data deposited in or computed by PubChem | |
InChI |
InChI=1S/C20H14O7/c21-12-7-8-13(22)19-18(12,27-19)16(23)15-17(24-15)20(19)25-10-5-1-3-9-4-2-6-11(26-20)14(9)10/h1-8,12,15-17,21,23H/t12-,15+,16-,17+,18-,19-/m0/s1 | |
Source | PubChem | |
URL | https://pubchem.ncbi.nlm.nih.gov | |
Description | Data deposited in or computed by PubChem | |
InChI Key |
AUWGMDYISSBOED-CCNMWVGKSA-N | |
Source | PubChem | |
URL | https://pubchem.ncbi.nlm.nih.gov | |
Description | Data deposited in or computed by PubChem | |
Canonical SMILES |
C1=CC2=C3C(=C1)OC4(C5C(O5)C(C67C4(O6)C(=O)C=CC7O)O)OC3=CC=C2 | |
Source | PubChem | |
URL | https://pubchem.ncbi.nlm.nih.gov | |
Description | Data deposited in or computed by PubChem | |
Isomeric SMILES |
C1=CC2=C3C(=C1)OC4([C@H]5[C@H](O5)[C@@H]([C@@]67[C@@]4(O6)C(=O)C=C[C@@H]7O)O)OC3=CC=C2 | |
Source | PubChem | |
URL | https://pubchem.ncbi.nlm.nih.gov | |
Description | Data deposited in or computed by PubChem | |
Molecular Formula |
C20H14O7 | |
Source | PubChem | |
URL | https://pubchem.ncbi.nlm.nih.gov | |
Description | Data deposited in or computed by PubChem | |
Molecular Weight |
366.3 g/mol | |
Source | PubChem | |
URL | https://pubchem.ncbi.nlm.nih.gov | |
Description | Data deposited in or computed by PubChem | |
Retrosynthesis Analysis
AI-Powered Synthesis Planning: Our tool employs the Template_relevance Pistachio, Template_relevance Bkms_metabolic, Template_relevance Pistachio_ringbreaker, Template_relevance Reaxys, Template_relevance Reaxys_biocatalysis model, leveraging a vast database of chemical reactions to predict feasible synthetic routes.
One-Step Synthesis Focus: Specifically designed for one-step synthesis, it provides concise and direct routes for your target compounds, streamlining the synthesis process.
Accurate Predictions: Utilizing the extensive PISTACHIO, BKMS_METABOLIC, PISTACHIO_RINGBREAKER, REAXYS, REAXYS_BIOCATALYSIS database, our tool offers high-accuracy predictions, reflecting the latest in chemical research and data.
Strategy Settings
Precursor scoring | Relevance Heuristic |
---|---|
Min. plausibility | 0.01 |
Model | Template_relevance |
Template Set | Pistachio/Bkms_metabolic/Pistachio_ringbreaker/Reaxys/Reaxys_biocatalysis |
Top-N result to add to graph | 6 |
Feasible Synthetic Routes
試験管内研究製品の免責事項と情報
BenchChemで提示されるすべての記事および製品情報は、情報提供を目的としています。BenchChemで購入可能な製品は、生体外研究のために特別に設計されています。生体外研究は、ラテン語の "in glass" に由来し、生物体の外で行われる実験を指します。これらの製品は医薬品または薬として分類されておらず、FDAから任何の医療状態、病気、または疾患の予防、治療、または治癒のために承認されていません。これらの製品を人間または動物に体内に導入する形態は、法律により厳格に禁止されています。これらのガイドラインに従うことは、研究と実験において法的および倫理的な基準の遵守を確実にするために重要です。