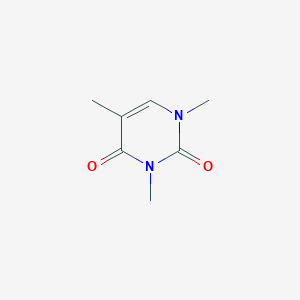
1,3-ジメチルチミン
概要
説明
1,3-Dimethylthymine is an organic compound with the molecular formula C₇H₁₀N₂O₂. It is a derivative of thymine, a pyrimidine nucleobase found in DNA. This compound is characterized by the presence of two methyl groups attached to the nitrogen atoms at positions 1 and 3 of the thymine ring. It is a colorless solid that is stable at room temperature and soluble in organic solvents such as anhydrous alcohol, ether, and chloroform .
科学的研究の応用
1,3-Dimethylthymine has several applications in scientific research:
Chemistry: It is used as a model compound in studies of nucleobase chemistry and photochemistry.
Biology: Research involving 1,3-Dimethylthymine helps in understanding DNA interactions and the effects of methylation on nucleobase stability and function.
Medicine: It serves as a reference compound in the development of drugs targeting DNA and RNA, particularly in the context of cancer research and antiviral therapies.
作用機序
Target of Action
1,3-Dimethylthymine is a derivative of thymine, a nucleobase found in DNA. The primary targets of 1,3-Dimethylthymine are likely to be similar to those of thymine, which include DNA polymerases and other enzymes involved in DNA replication and repair . .
Mode of Action
The mode of action of 1,3-Dimethylthymine is not well-studied. As a thymine derivative, it may interact with its targets in a similar manner to thymine, participating in hydrogen bonding with adenine during DNA replication. The additional methyl groups could potentially alter its interactions with enzymes and other biomolecules .
Biochemical Pathways
The specific biochemical pathways affected by 1,3-Dimethylthymine are not well-documented. Given its structural similarity to thymine, it may be involved in pathways related to DNA replication and repair. The impact of the additional methyl groups on these pathways is unclear .
Pharmacokinetics
Information on the pharmacokinetics of 1,3-Dimethylthymine is limited. The compound’s lipophilicity, as indicated by its Log Kow value of 0.10 , could influence its absorption and distribution .
Result of Action
The molecular and cellular effects of 1,3-Dimethylthymine’s action are not well-understood. Its structural similarity to thymine suggests it may incorporate into DNA and potentially cause mutations or other alterations in DNA structure. Specific studies on these effects are lacking .
Action Environment
The action of 1,3-Dimethylthymine could be influenced by various environmental factors. For instance, its stability and efficacy could be affected by pH, temperature, and the presence of other biomolecules. Additionally, its interaction with carbon radicals has been investigated , suggesting that it may participate in redox reactions and potentially have antioxidant properties .
準備方法
1,3-Dimethylthymine can be synthesized through various synthetic routes. One common method involves the methylation of thymine using methyl iodide in the presence of a base such as sodium hydride. The reaction typically occurs in an anhydrous solvent like dimethylformamide under reflux conditions . Industrial production methods may involve similar methylation reactions but on a larger scale, utilizing continuous flow reactors to enhance efficiency and yield.
化学反応の分析
1,3-Dimethylthymine undergoes several types of chemical reactions, including:
Oxidation: It can be oxidized using strong oxidizing agents like potassium permanganate or chromium trioxide, leading to the formation of various oxidation products.
Reduction: Reduction reactions can be carried out using reducing agents such as lithium aluminum hydride, resulting in the formation of reduced derivatives.
Substitution: Nucleophilic substitution reactions can occur at the methyl groups, especially under basic conditions.
Major products formed from these reactions depend on the specific conditions and reagents used. For example, oxidation may yield carboxylic acids, while reduction can produce alcohols or amines.
類似化合物との比較
1,3-Dimethylthymine is similar to other methylated nucleobases such as:
1,3-Dimethyluracil: Another pyrimidine derivative with similar methylation patterns but differing in the absence of a methyl group at position 5.
Caffeine: A purine derivative with multiple methyl groups, known for its stimulant properties.
Thymine: The parent compound, which lacks the additional methyl groups at positions 1 and 3
The uniqueness of 1,3-Dimethylthymine lies in its specific methylation pattern, which influences its chemical reactivity and interactions with nucleic acids, making it a valuable compound in various research and industrial applications.
特性
IUPAC Name |
1,3,5-trimethylpyrimidine-2,4-dione | |
---|---|---|
Source | PubChem | |
URL | https://pubchem.ncbi.nlm.nih.gov | |
Description | Data deposited in or computed by PubChem | |
InChI |
InChI=1S/C7H10N2O2/c1-5-4-8(2)7(11)9(3)6(5)10/h4H,1-3H3 | |
Source | PubChem | |
URL | https://pubchem.ncbi.nlm.nih.gov | |
Description | Data deposited in or computed by PubChem | |
InChI Key |
BHWQEVXICGSHEW-UHFFFAOYSA-N | |
Source | PubChem | |
URL | https://pubchem.ncbi.nlm.nih.gov | |
Description | Data deposited in or computed by PubChem | |
Canonical SMILES |
CC1=CN(C(=O)N(C1=O)C)C | |
Source | PubChem | |
URL | https://pubchem.ncbi.nlm.nih.gov | |
Description | Data deposited in or computed by PubChem | |
Molecular Formula |
C7H10N2O2 | |
Source | PubChem | |
URL | https://pubchem.ncbi.nlm.nih.gov | |
Description | Data deposited in or computed by PubChem | |
DSSTOX Substance ID |
DTXSID60196014 | |
Record name | N,N-Dimethylthymine | |
Source | EPA DSSTox | |
URL | https://comptox.epa.gov/dashboard/DTXSID60196014 | |
Description | DSSTox provides a high quality public chemistry resource for supporting improved predictive toxicology. | |
Molecular Weight |
154.17 g/mol | |
Source | PubChem | |
URL | https://pubchem.ncbi.nlm.nih.gov | |
Description | Data deposited in or computed by PubChem | |
CAS No. |
4401-71-2 | |
Record name | N,N-Dimethylthymine | |
Source | ChemIDplus | |
URL | https://pubchem.ncbi.nlm.nih.gov/substance/?source=chemidplus&sourceid=0004401712 | |
Description | ChemIDplus is a free, web search system that provides access to the structure and nomenclature authority files used for the identification of chemical substances cited in National Library of Medicine (NLM) databases, including the TOXNET system. | |
Record name | N,N-Dimethylthymine | |
Source | EPA DSSTox | |
URL | https://comptox.epa.gov/dashboard/DTXSID60196014 | |
Description | DSSTox provides a high quality public chemistry resource for supporting improved predictive toxicology. | |
Retrosynthesis Analysis
AI-Powered Synthesis Planning: Our tool employs the Template_relevance Pistachio, Template_relevance Bkms_metabolic, Template_relevance Pistachio_ringbreaker, Template_relevance Reaxys, Template_relevance Reaxys_biocatalysis model, leveraging a vast database of chemical reactions to predict feasible synthetic routes.
One-Step Synthesis Focus: Specifically designed for one-step synthesis, it provides concise and direct routes for your target compounds, streamlining the synthesis process.
Accurate Predictions: Utilizing the extensive PISTACHIO, BKMS_METABOLIC, PISTACHIO_RINGBREAKER, REAXYS, REAXYS_BIOCATALYSIS database, our tool offers high-accuracy predictions, reflecting the latest in chemical research and data.
Strategy Settings
Precursor scoring | Relevance Heuristic |
---|---|
Min. plausibility | 0.01 |
Model | Template_relevance |
Template Set | Pistachio/Bkms_metabolic/Pistachio_ringbreaker/Reaxys/Reaxys_biocatalysis |
Top-N result to add to graph | 6 |
Feasible Synthetic Routes
Q1: What is the molecular formula and weight of 1,3-Dimethylthymine?
A1: The molecular formula of 1,3-Dimethylthymine is C7H10N2O2, and its molecular weight is 154.17 g/mol.
Q2: Is there any spectroscopic data available for 1,3-Dimethylthymine?
A2: While the provided research papers don't offer comprehensive spectroscopic data, they utilize techniques like UV-absorption, fluorescence, NMR, and electron spin resonance (ESR) spectroscopy to study DMT's reactions and products. [, , , ]
Q3: How does 1,3-Dimethylthymine react with hydroxyl radicals?
A3: Research indicates that hydroxyl radicals primarily attack the C(6) position of the 1,3-Dimethylthymine molecule. This contrasts with thymine, where attack is favored at the C(5) position. []
Q4: Can 1,3-Dimethylthymine undergo photochemical reactions with other molecules?
A4: Yes, DMT participates in [2+2] photocycloadditions with compounds like benzophenone and its derivatives (Paternò-Büchi reaction). These reactions generate regioisomeric oxetanes, with the regioselectivity influenced by substituents on the benzophenone molecule and temperature. [, , , ]
Q5: How does temperature affect the regioselectivity of the Paternò-Büchi reaction with 1,3-Dimethylthymine?
A5: Studies reveal a significant temperature dependence on the regioselectivity. As temperature increases, the yield of one regioisomer decreases while the other increases. This suggests a change in the selectivity-determining step, potentially involving triplet 1,4-diradicals as intermediates. []
Q6: Does 1,3-Dimethylthymine react differently with SO4•– compared to other pyrimidine bases?
A6: Yes, unlike other pyrimidines like uracil, DMT reacts with sulfate radical anions (SO4•–) to primarily form C(6)-OH adduct radicals. Additionally, DMT does not form phosphate adduct radicals with SO4•– and phosphate mixtures or peroxodiphosphate. []
Q7: Can 1,3-Dimethylthymine undergo free radical alkylation?
A7: Yes, reactions with benzoyl peroxide in various solvents like cyclohexane, ethyl acetate, and acetonitrile can lead to free radical alkylation at the C(5) position. [, ]
Q8: How does the presence of sodium peroxodisulfate affect 1,3-Dimethylthymine?
A8: Heating DMT in the presence of sodium peroxodisulfate leads to oxidation, yielding 5-hydroxy-1,3-dimethyluracil as the primary product. []
Q9: Have computational methods been used to study 1,3-Dimethylthymine?
A9: Yes, computational studies, including CASSCF/CASPT2 calculations, have been employed to understand the mechanism of photoinduced cycloreversion of DMT-containing oxetanes. These studies provide insights into the energy barriers and intermediates involved in the reaction pathway. []
Q10: Does 1,3-Dimethylthymine play a role in DNA damage and repair?
A10: While not naturally present in DNA, DMT serves as a model compound to study thymine reactivity and DNA damage. Its reactions with radicals and photochemical processes help understand the potential pathways of DNA damage and repair mechanisms. [, , , , , ]
Q11: Can 1,3-Dimethylthymine be used to study the (6-4) photoproduct photolyase enzyme?
A11: DMT has been used to create model compounds for the oxetane intermediate in (6-4) photoproduct DNA lesion repair. These models help investigate the photorepair mechanism, particularly the role of electron transfer and cycloreversion in restoring the original bases. []
試験管内研究製品の免責事項と情報
BenchChemで提示されるすべての記事および製品情報は、情報提供を目的としています。BenchChemで購入可能な製品は、生体外研究のために特別に設計されています。生体外研究は、ラテン語の "in glass" に由来し、生物体の外で行われる実験を指します。これらの製品は医薬品または薬として分類されておらず、FDAから任何の医療状態、病気、または疾患の予防、治療、または治癒のために承認されていません。これらの製品を人間または動物に体内に導入する形態は、法律により厳格に禁止されています。これらのガイドラインに従うことは、研究と実験において法的および倫理的な基準の遵守を確実にするために重要です。