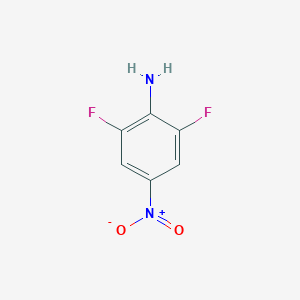
2,6-ジフルオロ-4-ニトロアニリン
概要
説明
2,6-Difluoro-4-nitroaniline is a chemical compound with the molecular formula C6H4F2N2O2 . It has a molecular weight of 174.11 and is a solid at room temperature . The compound is typically stored in a dark place under an inert atmosphere at temperatures between 2-8°C .
Molecular Structure Analysis
The molecular structure of 2,6-Difluoro-4-nitroaniline consists of a benzene ring substituted with two fluorine atoms, one nitro group, and one amino group . The InChI code for this compound is 1S/C6H4F2N2O2/c7-4-1-3 (10 (11)12)2-5 (8)6 (4)9/h1-2H,9H2 .Physical And Chemical Properties Analysis
2,6-Difluoro-4-nitroaniline is a solid at room temperature . It is typically stored in a dark place under an inert atmosphere at temperatures between 2-8°C .科学的研究の応用
化学合成
2,6-ジフルオロ-4-ニトロアニリンは化学合成に使用されます . 分子量は174.11であり、通常2〜8°Cで保管されます . 室温では固体です .
光学用途
2,4-ジニトロアニリンと同様に、2,6-ジフルオロ-4-ニトロアニリンは光学用途に使用される可能性があります . 有機アニリン構造は、多くの医薬品、電気光学、およびその他の社会的な用途における価値から、非常に研究されています . 有機材料は、UV-可視領域で良好な光学的非線形性、化学的柔軟性、熱安定性、および優れた透過率を持っています .
非線形光学材料
有機非線形光学(NLO)材料は、レーザー技術、発光ダイオード、トランジスタ、導体、電子材料、光学回路、光通信、光データストレージ技術、光信号処理、カラーディスプレイ、倍波、集積光学、フォトニック用途、電気光学変調器、および電気光学スイッチで使用されます .
単結晶成長
2,6-ジフルオロ-4-ニトロアニリンは、単結晶の成長に使用される可能性があります . 成長した結晶は、単結晶XRD、粉末XRD、FTIRおよびFT-ラマン分光法、光学、熱、誘電率、および3次非線形光学分析によって特徴付けることができます .
農薬検出
Safety and Hazards
2,6-Difluoro-4-nitroaniline is classified as a hazardous substance . It is harmful if swallowed, in contact with skin, or if inhaled . It is recommended to avoid breathing dust, fume, gas, mist, vapors, or spray . Protective gloves, protective clothing, eye protection, and face protection should be worn when handling this compound .
作用機序
Target of Action
Aromatic amines, such as 2,6-difluoro-4-nitroaniline, are known to interact with various substrates in chemical synthesis, production of polymers, and other industrial applications .
Mode of Action
It’s known that the amino group in anilines plays a crucial role in most interactions with different substrates . The presence of the nitro group and the fluorine atoms may influence the compound’s reactivity and interaction with its targets.
Pharmacokinetics
It’s predicted to have high gastrointestinal absorption and be bbb permeant . Its lipophilicity (Log Po/w) is estimated to be around 1.01 , which could influence its distribution and bioavailability.
Action Environment
The action of 2,6-Difluoro-4-nitroaniline can be influenced by various environmental factors. For instance, solvent models were used in a theoretical investigation to study the influence of the media on the electronic structure and geometry of the molecule . It was found that calculations using solvent models could obtain more accurate results than for vacuum .
特性
IUPAC Name |
2,6-difluoro-4-nitroaniline | |
---|---|---|
Source | PubChem | |
URL | https://pubchem.ncbi.nlm.nih.gov | |
Description | Data deposited in or computed by PubChem | |
InChI |
InChI=1S/C6H4F2N2O2/c7-4-1-3(10(11)12)2-5(8)6(4)9/h1-2H,9H2 | |
Source | PubChem | |
URL | https://pubchem.ncbi.nlm.nih.gov | |
Description | Data deposited in or computed by PubChem | |
InChI Key |
WDFFWUVELIFAOP-UHFFFAOYSA-N | |
Source | PubChem | |
URL | https://pubchem.ncbi.nlm.nih.gov | |
Description | Data deposited in or computed by PubChem | |
Canonical SMILES |
C1=C(C=C(C(=C1F)N)F)[N+](=O)[O-] | |
Source | PubChem | |
URL | https://pubchem.ncbi.nlm.nih.gov | |
Description | Data deposited in or computed by PubChem | |
Molecular Formula |
C6H4F2N2O2 | |
Source | PubChem | |
URL | https://pubchem.ncbi.nlm.nih.gov | |
Description | Data deposited in or computed by PubChem | |
DSSTOX Substance ID |
DTXSID70378925 | |
Record name | 2,6-Difluoro-4-nitroaniline | |
Source | EPA DSSTox | |
URL | https://comptox.epa.gov/dashboard/DTXSID70378925 | |
Description | DSSTox provides a high quality public chemistry resource for supporting improved predictive toxicology. | |
Molecular Weight |
174.10 g/mol | |
Source | PubChem | |
URL | https://pubchem.ncbi.nlm.nih.gov | |
Description | Data deposited in or computed by PubChem | |
CAS RN |
23156-27-6 | |
Record name | 2,6-Difluoro-4-nitroaniline | |
Source | EPA DSSTox | |
URL | https://comptox.epa.gov/dashboard/DTXSID70378925 | |
Description | DSSTox provides a high quality public chemistry resource for supporting improved predictive toxicology. | |
Record name | 2,6-Difluoro-4-nitroaniline | |
Source | European Chemicals Agency (ECHA) | |
URL | https://echa.europa.eu/information-on-chemicals | |
Description | The European Chemicals Agency (ECHA) is an agency of the European Union which is the driving force among regulatory authorities in implementing the EU's groundbreaking chemicals legislation for the benefit of human health and the environment as well as for innovation and competitiveness. | |
Explanation | Use of the information, documents and data from the ECHA website is subject to the terms and conditions of this Legal Notice, and subject to other binding limitations provided for under applicable law, the information, documents and data made available on the ECHA website may be reproduced, distributed and/or used, totally or in part, for non-commercial purposes provided that ECHA is acknowledged as the source: "Source: European Chemicals Agency, http://echa.europa.eu/". Such acknowledgement must be included in each copy of the material. ECHA permits and encourages organisations and individuals to create links to the ECHA website under the following cumulative conditions: Links can only be made to webpages that provide a link to the Legal Notice page. | |
Retrosynthesis Analysis
AI-Powered Synthesis Planning: Our tool employs the Template_relevance Pistachio, Template_relevance Bkms_metabolic, Template_relevance Pistachio_ringbreaker, Template_relevance Reaxys, Template_relevance Reaxys_biocatalysis model, leveraging a vast database of chemical reactions to predict feasible synthetic routes.
One-Step Synthesis Focus: Specifically designed for one-step synthesis, it provides concise and direct routes for your target compounds, streamlining the synthesis process.
Accurate Predictions: Utilizing the extensive PISTACHIO, BKMS_METABOLIC, PISTACHIO_RINGBREAKER, REAXYS, REAXYS_BIOCATALYSIS database, our tool offers high-accuracy predictions, reflecting the latest in chemical research and data.
Strategy Settings
Precursor scoring | Relevance Heuristic |
---|---|
Min. plausibility | 0.01 |
Model | Template_relevance |
Template Set | Pistachio/Bkms_metabolic/Pistachio_ringbreaker/Reaxys/Reaxys_biocatalysis |
Top-N result to add to graph | 6 |
Feasible Synthetic Routes
Q & A
Q1: What is the significance of studying 2,6-Difluoro-4-nitroaniline using computational methods?
A1: Understanding the electronic structure and geometry of molecules like 2,6-Difluoro-4-nitroaniline is crucial for predicting their reactivity and behavior in different environments. The research aimed to investigate these properties using semi-empirical computational methods []. This approach offers a cost-effective way to gain insights into the molecule's properties compared to more computationally demanding methods.
Q2: How does the presence of a solvent affect the electronic structure and geometry of 2,6-Difluoro-4-nitroaniline?
A2: The study employed both protic and aprotic Polarized Continuum solvent models to simulate the effect of different solvents []. The results indicated that incorporating solvent models into the calculations led to more accurate predictions compared to calculations performed in vacuum. This highlights the importance of considering environmental factors when studying molecular properties.
Q3: How well do the computational predictions align with experimental data for 2,6-Difluoro-4-nitroaniline?
A3: The researchers compared their calculated geometric parameters with experimental data obtained from X-ray diffraction (XRD) crystallography []. They found that the calculated values were within acceptable deviations from the experimental data, indicating the reliability and accuracy of the chosen computational methods for studying this molecule.
試験管内研究製品の免責事項と情報
BenchChemで提示されるすべての記事および製品情報は、情報提供を目的としています。BenchChemで購入可能な製品は、生体外研究のために特別に設計されています。生体外研究は、ラテン語の "in glass" に由来し、生物体の外で行われる実験を指します。これらの製品は医薬品または薬として分類されておらず、FDAから任何の医療状態、病気、または疾患の予防、治療、または治癒のために承認されていません。これらの製品を人間または動物に体内に導入する形態は、法律により厳格に禁止されています。これらのガイドラインに従うことは、研究と実験において法的および倫理的な基準の遵守を確実にするために重要です。