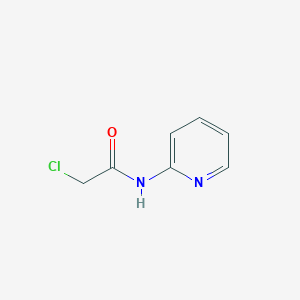
2-chloro-N-pyridin-2-ylacetamide
概要
説明
2-chloro-N-pyridin-2-ylacetamide is a chemical compound with the molecular formula C7H7ClN2O and a molecular weight of 170.6 g/mol . It is characterized by the presence of a chloro group attached to an acetamide moiety, which is further connected to a pyridine ring. This compound is known for its light-red to brown solid form and is used in various chemical and industrial applications .
準備方法
Synthetic Routes and Reaction Conditions
The synthesis of 2-chloro-N-pyridin-2-ylacetamide typically involves the reaction of 2-chloropyridine with acetamide under specific conditions. One common method includes the use of a base such as sodium hydroxide or potassium carbonate to facilitate the reaction. The reaction is usually carried out in an organic solvent like dimethylformamide (DMF) or dimethyl sulfoxide (DMSO) at elevated temperatures .
Industrial Production Methods
In industrial settings, the production of this compound may involve more scalable and efficient processes. These methods often utilize continuous flow reactors and optimized reaction conditions to ensure high yield and purity. The use of catalysts and advanced purification techniques such as recrystallization or chromatography may also be employed to achieve the desired product quality .
化学反応の分析
Types of Reactions
2-chloro-N-pyridin-2-ylacetamide undergoes various chemical reactions, including:
Substitution Reactions: The chloro group can be substituted with other nucleophiles such as amines, thiols, or alkoxides.
Oxidation Reactions: The compound can be oxidized to form corresponding N-oxides or other oxidized derivatives.
Reduction Reactions: Reduction of the compound can lead to the formation of amines or other reduced products.
Common Reagents and Conditions
Substitution: Reagents like sodium azide, potassium thiolate, or sodium alkoxide in solvents like ethanol or methanol.
Oxidation: Oxidizing agents such as hydrogen peroxide, m-chloroperbenzoic acid (m-CPBA), or potassium permanganate.
Major Products Formed
Substitution: Formation of N-substituted acetamides.
Oxidation: Formation of N-oxides or other oxidized derivatives.
Reduction: Formation of amines or other reduced products.
科学的研究の応用
2-chloro-N-pyridin-2-ylacetamide has a wide range of applications in scientific research:
Chemistry: Used as an intermediate in the synthesis of various organic compounds and pharmaceuticals.
Biology: Employed in the study of enzyme inhibition and protein-ligand interactions.
Medicine: Investigated for its potential therapeutic properties, including antimicrobial and anticancer activities.
Industry: Utilized in the production of agrochemicals, dyes, and other specialty chemicals.
作用機序
The mechanism of action of 2-chloro-N-pyridin-2-ylacetamide involves its interaction with specific molecular targets. In biological systems, it may act as an inhibitor of certain enzymes or receptors, thereby modulating various biochemical pathways. The chloro group and pyridine ring play crucial roles in its binding affinity and specificity towards these targets .
類似化合物との比較
Similar Compounds
- 2-chloro-N-methylacetamide
- 2-chloro-N-phenylacetamide
- 2-chloro-N-(2-pyridyl)acetamide
Uniqueness
2-chloro-N-pyridin-2-ylacetamide is unique due to the presence of the pyridine ring, which imparts distinct electronic and steric properties. This uniqueness allows it to exhibit specific reactivity patterns and biological activities that are different from other similar compounds .
特性
IUPAC Name |
2-chloro-N-pyridin-2-ylacetamide | |
---|---|---|
Source | PubChem | |
URL | https://pubchem.ncbi.nlm.nih.gov | |
Description | Data deposited in or computed by PubChem | |
InChI |
InChI=1S/C7H7ClN2O/c8-5-7(11)10-6-3-1-2-4-9-6/h1-4H,5H2,(H,9,10,11) | |
Source | PubChem | |
URL | https://pubchem.ncbi.nlm.nih.gov | |
Description | Data deposited in or computed by PubChem | |
InChI Key |
JJMRJPAPJCFDAM-UHFFFAOYSA-N | |
Source | PubChem | |
URL | https://pubchem.ncbi.nlm.nih.gov | |
Description | Data deposited in or computed by PubChem | |
Canonical SMILES |
C1=CC=NC(=C1)NC(=O)CCl | |
Source | PubChem | |
URL | https://pubchem.ncbi.nlm.nih.gov | |
Description | Data deposited in or computed by PubChem | |
Molecular Formula |
C7H7ClN2O | |
Source | PubChem | |
URL | https://pubchem.ncbi.nlm.nih.gov | |
Description | Data deposited in or computed by PubChem | |
DSSTOX Substance ID |
DTXSID50302480 | |
Record name | 2-Chloro-N-pyridin-2-yl-acetamide | |
Source | EPA DSSTox | |
URL | https://comptox.epa.gov/dashboard/DTXSID50302480 | |
Description | DSSTox provides a high quality public chemistry resource for supporting improved predictive toxicology. | |
Molecular Weight |
170.59 g/mol | |
Source | PubChem | |
URL | https://pubchem.ncbi.nlm.nih.gov | |
Description | Data deposited in or computed by PubChem | |
CAS No. |
5221-37-4 | |
Record name | 5221-37-4 | |
Source | DTP/NCI | |
URL | https://dtp.cancer.gov/dtpstandard/servlet/dwindex?searchtype=NSC&outputformat=html&searchlist=151214 | |
Description | The NCI Development Therapeutics Program (DTP) provides services and resources to the academic and private-sector research communities worldwide to facilitate the discovery and development of new cancer therapeutic agents. | |
Explanation | Unless otherwise indicated, all text within NCI products is free of copyright and may be reused without our permission. Credit the National Cancer Institute as the source. | |
Record name | 2-Chloro-N-pyridin-2-yl-acetamide | |
Source | EPA DSSTox | |
URL | https://comptox.epa.gov/dashboard/DTXSID50302480 | |
Description | DSSTox provides a high quality public chemistry resource for supporting improved predictive toxicology. | |
Synthesis routes and methods I
Procedure details
Synthesis routes and methods II
Procedure details
Synthesis routes and methods III
Procedure details
Retrosynthesis Analysis
AI-Powered Synthesis Planning: Our tool employs the Template_relevance Pistachio, Template_relevance Bkms_metabolic, Template_relevance Pistachio_ringbreaker, Template_relevance Reaxys, Template_relevance Reaxys_biocatalysis model, leveraging a vast database of chemical reactions to predict feasible synthetic routes.
One-Step Synthesis Focus: Specifically designed for one-step synthesis, it provides concise and direct routes for your target compounds, streamlining the synthesis process.
Accurate Predictions: Utilizing the extensive PISTACHIO, BKMS_METABOLIC, PISTACHIO_RINGBREAKER, REAXYS, REAXYS_BIOCATALYSIS database, our tool offers high-accuracy predictions, reflecting the latest in chemical research and data.
Strategy Settings
Precursor scoring | Relevance Heuristic |
---|---|
Min. plausibility | 0.01 |
Model | Template_relevance |
Template Set | Pistachio/Bkms_metabolic/Pistachio_ringbreaker/Reaxys/Reaxys_biocatalysis |
Top-N result to add to graph | 6 |
Feasible Synthetic Routes
試験管内研究製品の免責事項と情報
BenchChemで提示されるすべての記事および製品情報は、情報提供を目的としています。BenchChemで購入可能な製品は、生体外研究のために特別に設計されています。生体外研究は、ラテン語の "in glass" に由来し、生物体の外で行われる実験を指します。これらの製品は医薬品または薬として分類されておらず、FDAから任何の医療状態、病気、または疾患の予防、治療、または治癒のために承認されていません。これらの製品を人間または動物に体内に導入する形態は、法律により厳格に禁止されています。これらのガイドラインに従うことは、研究と実験において法的および倫理的な基準の遵守を確実にするために重要です。