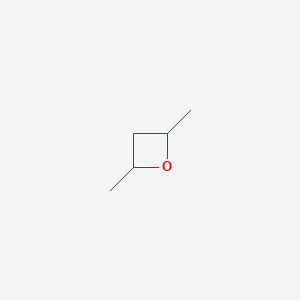
2,4-Dimethyloxetane
概要
説明
2,4-Dimethyloxetane is a cyclic ether with the molecular formula C₅H₁₀O. It is a four-membered ring compound with two methyl groups attached to the second and fourth carbon atoms. This compound is significant in various chemical processes, particularly in low-temperature combustion mechanisms where it acts as an intermediate .
準備方法
Synthetic Routes and Reaction Conditions
2,4-Dimethyloxetane can be synthesized through several methods, including:
Intramolecular Cyclization: This involves the cyclization of appropriate precursors under specific conditions.
Epoxide Ring Opening/Ring Closing: This method involves the opening of an epoxide ring followed by a ring-closing reaction to form the oxetane ring.
Industrial Production Methods
Industrial production of this compound typically involves the use of catalytic processes to ensure high yield and purity. The specific details of these processes are often proprietary, but they generally involve the use of metal catalysts and controlled reaction conditions to facilitate the cyclization reactions .
化学反応の分析
Types of Reactions
2,4-Dimethyloxetane undergoes various chemical reactions, including:
Reduction: This reaction involves the removal of oxygen or the addition of hydrogen to the compound.
Substitution: This reaction involves the replacement of one functional group with another.
Common Reagents and Conditions
Oxidation: Common reagents include oxygen (O₂) and hydrogen peroxide (H₂O₂).
Reduction: Common reagents include hydrogen gas (H₂) and metal catalysts such as palladium on carbon (Pd/C).
Substitution: Common reagents include halogens (e.g., chlorine, bromine) and nucleophiles (e.g., hydroxide ions).
Major Products
The major products formed from these reactions include various oxidized and reduced derivatives of this compound, as well as substituted oxetanes .
科学的研究の応用
2,4-Dimethyloxetane has several scientific research applications, including:
Chemistry: It is used as an intermediate in the synthesis of more complex organic compounds.
Biology: It is studied for its potential biological activity and interactions with biological molecules.
Medicine: It is explored for its potential use in drug development and as a building block for pharmaceuticals.
Industry: It is used in the production of polymers and other industrial chemicals.
作用機序
The mechanism of action of 2,4-dimethyloxetane involves its interaction with various molecular targets and pathways. For example, in combustion processes, it reacts with hydroperoxyalkyl radicals to form peroxy radicals, which then undergo further reactions to produce various products . The specific pathways and molecular targets depend on the reaction conditions and the presence of other reactants.
類似化合物との比較
2,4-Dimethyloxetane can be compared with other similar cyclic ethers, such as:
2-Methyloxetane: This compound has only one methyl group attached to the oxetane ring, making it less sterically hindered than this compound.
3,3-Dimethyloxetane: This compound has two methyl groups attached to the same carbon atom, resulting in different reactivity and steric effects compared to this compound.
The uniqueness of this compound lies in its specific substitution pattern, which influences its reactivity and the types of reactions it undergoes .
特性
IUPAC Name |
2,4-dimethyloxetane | |
---|---|---|
Source | PubChem | |
URL | https://pubchem.ncbi.nlm.nih.gov | |
Description | Data deposited in or computed by PubChem | |
InChI |
InChI=1S/C5H10O/c1-4-3-5(2)6-4/h4-5H,3H2,1-2H3 | |
Source | PubChem | |
URL | https://pubchem.ncbi.nlm.nih.gov | |
Description | Data deposited in or computed by PubChem | |
InChI Key |
KPPWZEMUMPFHEX-UHFFFAOYSA-N | |
Source | PubChem | |
URL | https://pubchem.ncbi.nlm.nih.gov | |
Description | Data deposited in or computed by PubChem | |
Canonical SMILES |
CC1CC(O1)C | |
Source | PubChem | |
URL | https://pubchem.ncbi.nlm.nih.gov | |
Description | Data deposited in or computed by PubChem | |
Molecular Formula |
C5H10O | |
Source | PubChem | |
URL | https://pubchem.ncbi.nlm.nih.gov | |
Description | Data deposited in or computed by PubChem | |
DSSTOX Substance ID |
DTXSID40336698 | |
Record name | Oxetane, 2,4-dimethyl- | |
Source | EPA DSSTox | |
URL | https://comptox.epa.gov/dashboard/DTXSID40336698 | |
Description | DSSTox provides a high quality public chemistry resource for supporting improved predictive toxicology. | |
Molecular Weight |
86.13 g/mol | |
Source | PubChem | |
URL | https://pubchem.ncbi.nlm.nih.gov | |
Description | Data deposited in or computed by PubChem | |
CAS No. |
14988-66-0 | |
Record name | Oxetane, 2,4-dimethyl- | |
Source | EPA DSSTox | |
URL | https://comptox.epa.gov/dashboard/DTXSID40336698 | |
Description | DSSTox provides a high quality public chemistry resource for supporting improved predictive toxicology. | |
Retrosynthesis Analysis
AI-Powered Synthesis Planning: Our tool employs the Template_relevance Pistachio, Template_relevance Bkms_metabolic, Template_relevance Pistachio_ringbreaker, Template_relevance Reaxys, Template_relevance Reaxys_biocatalysis model, leveraging a vast database of chemical reactions to predict feasible synthetic routes.
One-Step Synthesis Focus: Specifically designed for one-step synthesis, it provides concise and direct routes for your target compounds, streamlining the synthesis process.
Accurate Predictions: Utilizing the extensive PISTACHIO, BKMS_METABOLIC, PISTACHIO_RINGBREAKER, REAXYS, REAXYS_BIOCATALYSIS database, our tool offers high-accuracy predictions, reflecting the latest in chemical research and data.
Strategy Settings
Precursor scoring | Relevance Heuristic |
---|---|
Min. plausibility | 0.01 |
Model | Template_relevance |
Template Set | Pistachio/Bkms_metabolic/Pistachio_ringbreaker/Reaxys/Reaxys_biocatalysis |
Top-N result to add to graph | 6 |
Feasible Synthetic Routes
Q1: How is 2,4-dimethyloxetane formed during fuel combustion?
A1: this compound is a cyclic ether that forms during the low-temperature combustion of certain fuels, particularly n-pentane. [, ] Its formation is linked to the unimolecular reactions of hydroperoxyalkyl radicals (QOOH), specifically those derived from n-pentane. These QOOH radicals undergo an isomerization step involving a six-membered transition state, ultimately leading to the formation of this compound. []
Q2: Why is understanding the fate of this compound important in combustion modeling?
A2: this compound doesn't just sit inertly in the combustion environment. It can undergo further reactions that influence the overall reaction mechanism and product distribution. [] Critically, it can react via two main pathways:
- Unimolecular Ring-Opening: The oxetane ring can break apart, leading to various products depending on the specific radical formed and the reaction conditions. [] These ring-opening reactions have rates comparable to reactions with oxygen, making them significant competitors. []
- Reaction with Oxygen: this compound can react with molecular oxygen to form cyclic ether-peroxy adducts, further complicating the reaction network. []
Q3: What makes the study of this compound radicals complex?
A3: Several factors contribute to the complexity:
- Stereoisomerism: this compound can exist as different stereoisomers, and the stereochemistry of the molecule significantly affects the reactivity of its derived radicals. For instance, diastereomeric cyclic ether peroxy radicals (formed from this compound) exhibit vastly different reactivity profiles. []
- Multiple Reaction Channels: The potential energy surfaces of these radicals are complex, with multiple accessible reaction pathways leading to a diverse array of products. [, ] This includes conventional QOOH decomposition pathways (like HO2 elimination) and ring-opening reactions, which can lead to unique unsaturated peroxy radicals. []
- Well-Skipping Reactions: At atmospheric pressure, the rate of chemical reactions for many of these radicals is comparable to the rate of collisional stabilization. This leads to "well-skipping" reactions, where the energized radicals react before being fully thermalized, further complicating kinetic analysis. []
試験管内研究製品の免責事項と情報
BenchChemで提示されるすべての記事および製品情報は、情報提供を目的としています。BenchChemで購入可能な製品は、生体外研究のために特別に設計されています。生体外研究は、ラテン語の "in glass" に由来し、生物体の外で行われる実験を指します。これらの製品は医薬品または薬として分類されておらず、FDAから任何の医療状態、病気、または疾患の予防、治療、または治癒のために承認されていません。これらの製品を人間または動物に体内に導入する形態は、法律により厳格に禁止されています。これらのガイドラインに従うことは、研究と実験において法的および倫理的な基準の遵守を確実にするために重要です。