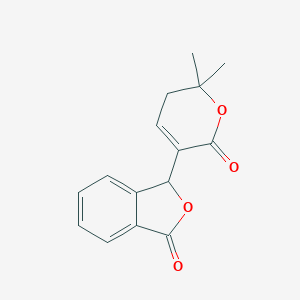
カタプラクトン
概要
説明
Catalpalactone is a dilactone compound that was first isolated from the heartwood of the ornamental tree Catalpa ovata G. Don, which is widely distributed across Korea, China, and Japan . It has also been found in the wood of a related species, Catalpa bignonioides Walt . Catalpalactone has been reported to exhibit various biological activities, including antitermitic, anti-inflammatory, and cytotoxic effects .
科学的研究の応用
Catalpalactone has a wide range of scientific research applications, including its use in chemistry, biology, medicine, and industry. In chemistry, it is used as a starting material for the synthesis of various derivatives with enhanced biological activities . In biology, catalpalactone has been studied for its antimicrobial, cytotoxic, and insecticidal properties . In medicine, it has shown potential as an anti-inflammatory agent by inhibiting nitric oxide production and inducible nitric oxide synthase expression in lipopolysaccharide-induced RAW264.7 cells . Additionally, catalpalactone has been investigated for its cytoprotective effects against oxidative damage in HepG2 cells .
作用機序
Target of Action
Catalpalactone, a dilactone isolated from the heartwood of the ornamental tree Catalpa ovata , primarily targets various types of cells and organisms. It exhibits antimicrobial activity against pathogens such as Escherichia coli, Staphylococcus aureus, and Micrococcus luteus . It also shows cytotoxic activities and insecticidal activities .
Mode of Action
Catalpalactone interacts with its targets through its α,β-unsaturated six-membered lactone ring . This structure is essential for its antimicrobial, cytotoxic, and insecticidal activities . In the context of anti-inflammatory effects, catalpalactone inhibits nitric oxide (NO) production and inducible NO synthase (iNOS) expression in lipopolysaccharide (LPS)-stimulated RAW264.7 cells .
Biochemical Pathways
Catalpalactone affects several biochemical pathways. It inhibits the production of nitric oxide (NO), a short-lived free radical synthesized by NO synthase (NOS) . It also suppresses the expression of inducible NO synthase (iNOS), which is expressed in response to LPS or pro-inflammatory cytokines . Furthermore, catalpalactone prevents the activation of interferon regulatory factor 3 (IRF3) and nuclear factor-κB (NF-κB), both of which are involved in the inflammatory response .
Pharmacokinetics
The pharmacokinetics of catalpalactone have been studied in Wistar rats . The study suggests that catalpalactone has superior pharmacokinetics, such as a longer half-life (T1/2) and mean residence time (MRT), lower clearance (CL), and better absorption in vivo . These properties make catalpalactone a promising candidate for further drug development and structure optimization .
Result of Action
Catalpalactone exhibits various effects at the molecular and cellular levels. It significantly inhibits nitric oxide (NO) production and inducible NO synthase (iNOS) expression in LPS-induced RAW264.7 cells . It also reduces the levels of cytokines such as interleukin-6 and tumor necrosis factor-α . Moreover, catalpalactone exerts cytoprotective effects against H2O2-induced oxidative damage in HepG2 cells .
Action Environment
The action of catalpalactone can be influenced by environmental factors. For instance, the methylene at the δ position of the α, β-unsaturated six-membered lactone ring, when replaced by bismethyl, causes a decrease or even loss of antifungal activities . This suggests that the chemical environment of catalpalactone can significantly affect its efficacy and stability.
Safety and Hazards
Catalpalactone is for R&D use only and not for medicinal, household, or other use . It is advised to avoid dust formation, breathing mist, gas, or vapors, and contacting with skin and eye . Use personal protective equipment, wear chemical impermeable gloves, ensure adequate ventilation, remove all sources of ignition, and evacuate personnel to safe areas .
生化学分析
Biochemical Properties
Catalpalactone plays a significant role in biochemical reactions. It interacts with various enzymes, proteins, and other biomolecules. For instance, it has been found to inhibit nitric oxide (NO) production and inducible NO synthase (iNOS) expression in lipopolysaccharide (LPS)-induced RAW264.7 cells .
Cellular Effects
Catalpalactone has profound effects on various types of cells and cellular processes. It influences cell function by impacting cell signaling pathways, gene expression, and cellular metabolism. In LPS-induced RAW264.7 cells, catalpalactone significantly inhibited nitric oxide (NO) production and inducible NO synthase (iNOS) expression .
Molecular Mechanism
The molecular mechanism of catalpalactone involves its interactions at the molecular level, including binding interactions with biomolecules, enzyme inhibition or activation, and changes in gene expression. It has been found to inhibit LPS-induced NO production and iNOS expression in RAW264.7 cells, and also inhibits IRF3, NF-κB, and IFN-β/STAT-1 activation .
準備方法
Synthetic Routes and Reaction Conditions: Catalpalactone can be synthesized through several synthetic routes. One common method involves the use of α,β-unsaturated six-member lactone rings, which are essential for its antimicrobial, cytotoxic, and insecticidal activities . The synthesis typically involves the use of reagents such as 1H NMR, 13C NMR, and HRMS to identify the structures of the synthesized compounds .
Industrial Production Methods: Industrial production of catalpalactone is not well-documented, but it is likely to involve similar synthetic routes as those used in laboratory settings. The process would require optimization for large-scale production, including the use of efficient catalysts and reaction conditions to maximize yield and purity.
化学反応の分析
Types of Reactions: Catalpalactone undergoes various chemical reactions, including oxidation, reduction, and substitution reactions. These reactions are influenced by the presence of the α,β-unsaturated six-member lactone ring, which is a key structural feature .
Common Reagents and Conditions: Common reagents used in the reactions of catalpalactone include nitric oxide, lipopolysaccharide, and hydrogen peroxide . Reaction conditions vary depending on the desired outcome, but typically involve controlled temperatures and pH levels to ensure optimal reaction rates and product formation.
Major Products Formed: The major products formed from the reactions of catalpalactone include antimicrobial, cytotoxic, and insecticidal compounds . These products have been evaluated for their biological activities and have shown promising results in various studies .
類似化合物との比較
Catalpalactone is unique due to its α,β-unsaturated six-member lactone ring, which is essential for its biological activities . Similar compounds include other dilactones and lactone-containing compounds that exhibit antimicrobial, cytotoxic, and insecticidal properties. Some of these similar compounds include:
- Catalpalactone derivatives (e.g., compounds d, g, h-4)
- Other lactone-containing compounds with similar biological activities
Catalpalactone stands out due to its specific structural features and the range of biological activities it exhibits, making it a valuable compound for further research and development.
特性
IUPAC Name |
3-(2,2-dimethyl-6-oxo-3H-pyran-5-yl)-3H-2-benzofuran-1-one | |
---|---|---|
Source | PubChem | |
URL | https://pubchem.ncbi.nlm.nih.gov | |
Description | Data deposited in or computed by PubChem | |
InChI |
InChI=1S/C15H14O4/c1-15(2)8-7-11(14(17)19-15)12-9-5-3-4-6-10(9)13(16)18-12/h3-7,12H,8H2,1-2H3 | |
Source | PubChem | |
URL | https://pubchem.ncbi.nlm.nih.gov | |
Description | Data deposited in or computed by PubChem | |
InChI Key |
GFYSRANGENPXDF-UHFFFAOYSA-N | |
Source | PubChem | |
URL | https://pubchem.ncbi.nlm.nih.gov | |
Description | Data deposited in or computed by PubChem | |
Canonical SMILES |
CC1(CC=C(C(=O)O1)C2C3=CC=CC=C3C(=O)O2)C | |
Source | PubChem | |
URL | https://pubchem.ncbi.nlm.nih.gov | |
Description | Data deposited in or computed by PubChem | |
Molecular Formula |
C15H14O4 | |
Source | PubChem | |
URL | https://pubchem.ncbi.nlm.nih.gov | |
Description | Data deposited in or computed by PubChem | |
DSSTOX Substance ID |
DTXSID10928201 | |
Record name | 3-(6,6-Dimethyl-2-oxo-5,6-dihydro-2H-pyran-3-yl)-2-benzofuran-1(3H)-one | |
Source | EPA DSSTox | |
URL | https://comptox.epa.gov/dashboard/DTXSID10928201 | |
Description | DSSTox provides a high quality public chemistry resource for supporting improved predictive toxicology. | |
Molecular Weight |
258.27 g/mol | |
Source | PubChem | |
URL | https://pubchem.ncbi.nlm.nih.gov | |
Description | Data deposited in or computed by PubChem | |
CAS No. |
1585-68-8, 133591-03-4 | |
Record name | Catalpalactone | |
Source | CAS Common Chemistry | |
URL | https://commonchemistry.cas.org/detail?cas_rn=1585-68-8 | |
Description | CAS Common Chemistry is an open community resource for accessing chemical information. Nearly 500,000 chemical substances from CAS REGISTRY cover areas of community interest, including common and frequently regulated chemicals, and those relevant to high school and undergraduate chemistry classes. This chemical information, curated by our expert scientists, is provided in alignment with our mission as a division of the American Chemical Society. | |
Explanation | The data from CAS Common Chemistry is provided under a CC-BY-NC 4.0 license, unless otherwise stated. | |
Record name | Catalpalactone | |
Source | ChemIDplus | |
URL | https://pubchem.ncbi.nlm.nih.gov/substance/?source=chemidplus&sourceid=0001585688 | |
Description | ChemIDplus is a free, web search system that provides access to the structure and nomenclature authority files used for the identification of chemical substances cited in National Library of Medicine (NLM) databases, including the TOXNET system. | |
Record name | 3-(6,6-Dimethyl-2-oxo-5,6-dihydro-2H-pyran-3-yl)-2-benzofuran-1(3H)-one | |
Source | EPA DSSTox | |
URL | https://comptox.epa.gov/dashboard/DTXSID10928201 | |
Description | DSSTox provides a high quality public chemistry resource for supporting improved predictive toxicology. | |
Record name | CATALPALACTONE | |
Source | FDA Global Substance Registration System (GSRS) | |
URL | https://gsrs.ncats.nih.gov/ginas/app/beta/substances/P61GH0V29Z | |
Description | The FDA Global Substance Registration System (GSRS) enables the efficient and accurate exchange of information on what substances are in regulated products. Instead of relying on names, which vary across regulatory domains, countries, and regions, the GSRS knowledge base makes it possible for substances to be defined by standardized, scientific descriptions. | |
Explanation | Unless otherwise noted, the contents of the FDA website (www.fda.gov), both text and graphics, are not copyrighted. They are in the public domain and may be republished, reprinted and otherwise used freely by anyone without the need to obtain permission from FDA. Credit to the U.S. Food and Drug Administration as the source is appreciated but not required. | |
Retrosynthesis Analysis
AI-Powered Synthesis Planning: Our tool employs the Template_relevance Pistachio, Template_relevance Bkms_metabolic, Template_relevance Pistachio_ringbreaker, Template_relevance Reaxys, Template_relevance Reaxys_biocatalysis model, leveraging a vast database of chemical reactions to predict feasible synthetic routes.
One-Step Synthesis Focus: Specifically designed for one-step synthesis, it provides concise and direct routes for your target compounds, streamlining the synthesis process.
Accurate Predictions: Utilizing the extensive PISTACHIO, BKMS_METABOLIC, PISTACHIO_RINGBREAKER, REAXYS, REAXYS_BIOCATALYSIS database, our tool offers high-accuracy predictions, reflecting the latest in chemical research and data.
Strategy Settings
Precursor scoring | Relevance Heuristic |
---|---|
Min. plausibility | 0.01 |
Model | Template_relevance |
Template Set | Pistachio/Bkms_metabolic/Pistachio_ringbreaker/Reaxys/Reaxys_biocatalysis |
Top-N result to add to graph | 6 |
Feasible Synthetic Routes
Q1: What is Catalpalactone and where is it found?
A1: Catalpalactone is a natural product first isolated from the wood of Catalpa ovata G.DON and C. bignonioides WALT. [] It is a unique phthalide derivative. []
Q2: What are the known biological activities of Catalpalactone?
A2: Catalpalactone has shown promising anti-inflammatory effects. In LPS-induced RAW264.7 cells (a model for inflammation), it significantly reduced nitric oxide (NO) production and suppressed the expression of iNOS (inducible NO synthase), a key enzyme involved in NO synthesis. [] Furthermore, it lowered the levels of pro-inflammatory cytokines like interleukin-6 and tumor necrosis factor-α in the same cellular model. [] Studies have also demonstrated Catalpalactone's inhibitory activity against TPA-induced Epstein-Barr virus early antigen (EBV-EA) activation in Raji cells, suggesting antitumor-promoting potential. []
Q3: How does Catalpalactone exert its anti-inflammatory effects?
A3: Research suggests that Catalpalactone's anti-inflammatory action stems from its ability to inhibit several key signaling pathways. It suppresses the activation of Nuclear Factor-κB (NF-κB) and Interferon Regulatory Factor 3 (IRF3), both crucial transcription factors involved in inflammatory responses. [] It also hinders the production of Interferon-β (IFN-β) and the expression of Signal Transducer and Activator of Transcription 1 (STAT-1) protein, further dampening the inflammatory cascade. []
Q4: What is the chemical structure of Catalpalactone?
A4: Catalpalactone is characterized by a unique phthalide framework fused with a butyrolactone ring. While its planar structure is well-established, the absolute configuration remains to be definitively elucidated. []
Q5: What are the key structural features of Catalpalactone important for its activity?
A5: While specific structure-activity relationship (SAR) studies are limited for Catalpalactone, its unique fused ring system, incorporating both a phthalide and a butyrolactone moiety, is likely crucial for its biological activity. Further investigations are needed to pinpoint the specific structural elements responsible for its interactions with biological targets and subsequent effects.
Q6: Have there been any attempts to synthesize Catalpalactone?
A6: Yes, several synthetic routes to Catalpalactone have been reported. One approach utilized a Wittig reaction followed by lactonization and selenolactonization steps. [] Other synthetic strategies have also been explored, aiming to improve the yield and efficiency of Catalpalactone synthesis. [, ]
Q7: What are the challenges in researching Catalpalactone further?
A7: Despite its promising bioactivities, research on Catalpalactone faces several challenges. These include:
Q8: Are there any known naphthoquinones found in the same plant species as Catalpalactone?
A9: Yes, various naphthoquinones have been isolated from Catalpa ovata, including α-lapachone, α-dihydrocaryopterone (9-hydroxy-α-lapachone), 9-methoxy-α-lapachone, 4-hydroxy-α-lapachone, 4,9-dihydroxy-α-lapachone, and 4-oxo-α-lapachone. [] Some of these compounds also exhibit biological activities, such as inhibitory effects on nitric oxide production. []
Q9: What is the significance of the co-occurrence of Catalpalactone and naphthoquinones in Catalpa ovata?
A10: The presence of both Catalpalactone and various naphthoquinones in Catalpa ovata suggests a potential biosynthetic link between these compounds. Further research into the biosynthetic pathways of these compounds within the plant could provide insights into their production and potential interrelationships. It may also uncover new bioactive molecules with potential therapeutic value. For instance, understanding the biosynthetic origin of the 2-carboxy-4-hydroxy-α-tetralone intermediate could shed light on the formation of both catalpalactones and 4,9-dihydroxy-α-lapachones. []
試験管内研究製品の免責事項と情報
BenchChemで提示されるすべての記事および製品情報は、情報提供を目的としています。BenchChemで購入可能な製品は、生体外研究のために特別に設計されています。生体外研究は、ラテン語の "in glass" に由来し、生物体の外で行われる実験を指します。これらの製品は医薬品または薬として分類されておらず、FDAから任何の医療状態、病気、または疾患の予防、治療、または治癒のために承認されていません。これらの製品を人間または動物に体内に導入する形態は、法律により厳格に禁止されています。これらのガイドラインに従うことは、研究と実験において法的および倫理的な基準の遵守を確実にするために重要です。