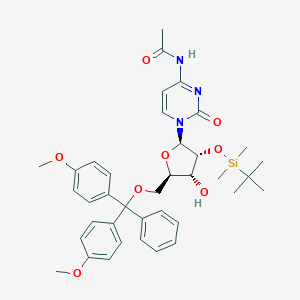
5'-O-DMT-2'-O-TBDMS-N-Ac-シチジン
- 専門家チームからの見積もりを受け取るには、QUICK INQUIRYをクリックしてください。
- 品質商品を競争力のある価格で提供し、研究に集中できます。
説明
5'-O-DMT-2'-O-TBDMS-Ac-rCは、DNAとRNAの合成に使用される修飾ヌクレオシドです。 ジメトキシトリチル(DMT)とtert-ブチルジメチルシリル(TBDMS)の保護基の存在が特徴であり、これは核酸合成中の安定性と機能性に不可欠です .
科学的研究の応用
5’-O-DMT-2’-O-TBDMS-Ac-rC is widely used in scientific research, particularly in the fields of:
Chemistry: As a building block for the synthesis of modified oligonucleotides
Biology: In the study of nucleic acid interactions and functions
Medicine: For the development of therapeutic oligonucleotides and gene therapy applications
Industry: In the production of synthetic RNA and DNA for various applications
作用機序
5'-O-DMT-2'-O-TBDMS-Ac-rCの作用機序には、合成中の核酸への組み込みが含まれます。 保護基(DMTとTBDMS)は、ヌクレオシドの安定性と、成長中のオリゴヌクレオチド鎖への適切な組み立てを保証します。 組み込まれると、保護基は除去され、ヌクレオシドが塩基対形成や核酸機能に不可欠な他の相互作用に参加できるようになります .
準備方法
合成経路と反応条件
5'-O-DMT-2'-O-TBDMS-Ac-rCの合成には、2'位と5'位のヒドロキシル基の保護から始まる複数の段階が含まれます。 2'-ヒドロキシル基はTBDMS基で保護され、5'-ヒドロキシル基はDMT基で保護されます。 アセチル基は、シチジン塩基のアミノ基を保護するために導入されます .
工業的生産方法
5'-O-DMT-2'-O-TBDMS-Ac-rCの工業的生産には、通常、自動化された固相合成技術が使用されます。 これらの方法は、化合物の効率的かつスケーラブルな生産を可能にし、高純度と収率を保証します .
化学反応の分析
反応の種類
5'-O-DMT-2'-O-TBDMS-Ac-rCは、以下を含むさまざまな化学反応を起こします。
脱保護反応: それぞれ酸性条件と塩基性条件下でのDMTとTBDMS基の除去.
置換反応: 保護されたヌクレオシドへの求核攻撃を含む.
一般的な試薬と条件
主な生成物
これらの反応から生成される主な生成物は、脱保護されたヌクレオシドであり、オリゴヌクレオチドの合成でさらに利用できます .
科学研究への応用
5'-O-DMT-2'-O-TBDMS-Ac-rCは、特に以下の分野で科学研究で広く使用されています。
類似化合物との比較
類似化合物
5'-O-DMT-2'-O-TBDMS-rU: 同様の保護基を持つ別の修飾ヌクレオシド.
5'-O-DMT-2'-O-TBDMS-rA: 修飾アデノシンヌクレオシド.
5'-O-DMT-2'-O-TBDMS-rG: 修飾グアノシンヌクレオシド.
独自性
5'-O-DMT-2'-O-TBDMS-Ac-rCは、保護基の特定の組み合わせと、シチジン含有オリゴヌクレオチドの合成への応用によって独自です。 これは、RNAベースの治療薬の研究と開発において特に価値があります .
生物活性
5-O-DMT-2-O-TBDMS-N-Ac-cytidine, also known as N-acetyl-5′-O-(4,4′-dimethoxytrityl)-2′-O-[(tert-butyldimethylsilyl)]-cytidine, is a modified nucleoside that plays a significant role in the synthesis of RNA and other nucleic acids. This compound is particularly valuable in organic chemistry and molecular biology due to its unique structural modifications that enhance its biological activity.
Chemical Structure and Properties
The molecular formula of 5-O-DMT-2-O-TBDMS-N-Ac-cytidine is C₃₈H₄₇N₃O₈Si, and it features:
- DMT Group : A dimethoxytrityl group at the 5' position.
- TBDMS Group : A tert-butyldimethylsilyl protecting group at the 2' position.
- Acetyl Modification : An N-acetyl modification on the cytosine base.
These modifications are crucial for improving the stability and reactivity of the nucleoside during RNA synthesis.
Biological Activity
The biological activity of 5-O-DMT-2-O-TBDMS-N-Ac-cytidine is primarily linked to its role as a building block in RNA synthesis. Modified nucleosides like this one can influence:
- Stability : Enhanced resistance to enzymatic degradation.
- Binding Affinity : Improved interactions with proteins and other nucleic acids, which are essential for therapeutic applications.
Table 1: Comparison of Biological Activities
Property | Standard Cytidine | 5-O-DMT-2-O-TBDMS-N-Ac-Cytidine |
---|---|---|
Stability against nucleases | Low | High |
Binding affinity | Moderate | Enhanced |
Resistance to hydrolysis | Low | High |
Application in RNA synthesis | Limited | Extensive |
Synthesis Methods
The synthesis of 5-O-DMT-2-O-TBDMS-N-Ac-cytidine typically involves several steps, including protection and deprotection processes. Recent advancements have introduced methods utilizing small organic catalysts that enhance yield and selectivity during these reactions. For instance, a study demonstrated high selectivity in producing related mononucleosides using organic catalysts, achieving yields up to 92% .
Case Studies and Research Findings
- RNA Therapeutics : Research has shown that modified nucleosides can significantly improve the pharmacokinetic properties of RNA-based therapeutics. A study highlighted how modifications at the 2' position enhance stability against enzymatic degradation while influencing hybridization properties with complementary strands .
- Interaction Studies : Various studies have focused on the interaction profiles of modified nucleosides with proteins and nucleic acids. These studies indicate that such modifications can lead to increased binding affinities, making them suitable candidates for therapeutic applications .
- Experimental Validation : In vitro assays have demonstrated that compounds similar to 5-O-DMT-2-O-TBDMS-N-Ac-cytidine exhibit enhanced binding affinity to target proteins compared to unmodified counterparts. This suggests potential applications in drug design and development .
特性
IUPAC Name |
N-[1-[(2R,3R,4R,5R)-5-[[bis(4-methoxyphenyl)-phenylmethoxy]methyl]-3-[tert-butyl(dimethyl)silyl]oxy-4-hydroxyoxolan-2-yl]-2-oxopyrimidin-4-yl]acetamide |
Source
|
---|---|---|
Source | PubChem | |
URL | https://pubchem.ncbi.nlm.nih.gov | |
Description | Data deposited in or computed by PubChem | |
InChI |
InChI=1S/C38H47N3O8Si/c1-25(42)39-32-22-23-41(36(44)40-32)35-34(49-50(7,8)37(2,3)4)33(43)31(48-35)24-47-38(26-12-10-9-11-13-26,27-14-18-29(45-5)19-15-27)28-16-20-30(46-6)21-17-28/h9-23,31,33-35,43H,24H2,1-8H3,(H,39,40,42,44)/t31-,33-,34-,35-/m1/s1 |
Source
|
Source | PubChem | |
URL | https://pubchem.ncbi.nlm.nih.gov | |
Description | Data deposited in or computed by PubChem | |
InChI Key |
ZFRWPAYPCFTQHK-HYGOWAQNSA-N |
Source
|
Source | PubChem | |
URL | https://pubchem.ncbi.nlm.nih.gov | |
Description | Data deposited in or computed by PubChem | |
Canonical SMILES |
CC(=O)NC1=NC(=O)N(C=C1)C2C(C(C(O2)COC(C3=CC=CC=C3)(C4=CC=C(C=C4)OC)C5=CC=C(C=C5)OC)O)O[Si](C)(C)C(C)(C)C |
Source
|
Source | PubChem | |
URL | https://pubchem.ncbi.nlm.nih.gov | |
Description | Data deposited in or computed by PubChem | |
Isomeric SMILES |
CC(=O)NC1=NC(=O)N(C=C1)[C@H]2[C@@H]([C@@H]([C@H](O2)COC(C3=CC=CC=C3)(C4=CC=C(C=C4)OC)C5=CC=C(C=C5)OC)O)O[Si](C)(C)C(C)(C)C |
Source
|
Source | PubChem | |
URL | https://pubchem.ncbi.nlm.nih.gov | |
Description | Data deposited in or computed by PubChem | |
Molecular Formula |
C38H47N3O8Si |
Source
|
Source | PubChem | |
URL | https://pubchem.ncbi.nlm.nih.gov | |
Description | Data deposited in or computed by PubChem | |
DSSTOX Substance ID |
DTXSID10456433 |
Source
|
Record name | N-Acetyl-5'-O-[bis(4-methoxyphenyl)(phenyl)methyl]-2'-O-[tert-butyl(dimethyl)silyl]cytidine | |
Source | EPA DSSTox | |
URL | https://comptox.epa.gov/dashboard/DTXSID10456433 | |
Description | DSSTox provides a high quality public chemistry resource for supporting improved predictive toxicology. | |
Molecular Weight |
701.9 g/mol |
Source
|
Source | PubChem | |
URL | https://pubchem.ncbi.nlm.nih.gov | |
Description | Data deposited in or computed by PubChem | |
CAS No. |
121058-85-3 |
Source
|
Record name | N-Acetyl-5'-O-[bis(4-methoxyphenyl)(phenyl)methyl]-2'-O-[tert-butyl(dimethyl)silyl]cytidine | |
Source | EPA DSSTox | |
URL | https://comptox.epa.gov/dashboard/DTXSID10456433 | |
Description | DSSTox provides a high quality public chemistry resource for supporting improved predictive toxicology. | |
試験管内研究製品の免責事項と情報
BenchChemで提示されるすべての記事および製品情報は、情報提供を目的としています。BenchChemで購入可能な製品は、生体外研究のために特別に設計されています。生体外研究は、ラテン語の "in glass" に由来し、生物体の外で行われる実験を指します。これらの製品は医薬品または薬として分類されておらず、FDAから任何の医療状態、病気、または疾患の予防、治療、または治癒のために承認されていません。これらの製品を人間または動物に体内に導入する形態は、法律により厳格に禁止されています。これらのガイドラインに従うことは、研究と実験において法的および倫理的な基準の遵守を確実にするために重要です。