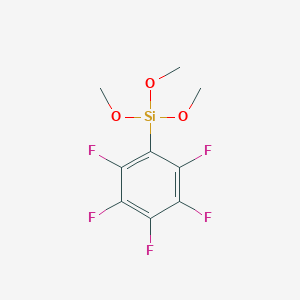
トリメトキシ(ペンタフルオロフェニル)シラン
概要
説明
Trimethoxy(pentafluorophenyl)silane is an organosilicon compound with the molecular formula C9H9F5O3Si. It is characterized by the presence of a pentafluorophenyl group attached to a silicon atom, which is further bonded to three methoxy groups. This compound is known for its reactivity and is used in various chemical processes and applications.
科学的研究の応用
Trimethoxy(pentafluorophenyl)silane has a wide range of applications in scientific research, including:
Chemistry: It is used as a reagent in organic synthesis, particularly in the formation of carbon-silicon bonds and in cross-coupling reactions.
Biology: The compound can be used to modify surfaces of biological materials, such as proteins or cells, to study their interactions and properties.
Industry: The compound is used in the production of advanced materials, such as coatings and adhesives, where its reactivity and stability are advantageous.
作用機序
Target of Action
Trimethoxy(pentafluorophenyl)silane is an organosilicon compound . It is primarily used as a reagent in organic synthesis . Its primary targets are organic compounds that can undergo reactions catalyzed by transition metals .
Mode of Action
Trimethoxy(pentafluorophenyl)silane acts as a silicon-based nucleophile . It is reactive in palladium-catalyzed cross-coupling reactions . This means it donates an electron pair to form a chemical bond during these reactions.
Biochemical Pathways
The specific biochemical pathways affected by Trimethoxy(pentafluorophenyl)silane depend on the organic compounds it is reacting with. In general, it participates in reactions that form carbon-carbon and carbon-silicon bonds . These reactions are fundamental to the synthesis of complex organic molecules.
Pharmacokinetics
Its physical properties such as its boiling point (1845±400 °C) and density (1373 g/cm³) can impact its handling and use in chemical reactions .
Result of Action
The result of Trimethoxy(pentafluorophenyl)silane’s action is the formation of new organic compounds via the creation of carbon-carbon and carbon-silicon bonds . This can lead to the synthesis of complex organic molecules, which have a wide range of applications in fields such as pharmaceuticals, materials science, and more .
準備方法
Synthetic Routes and Reaction Conditions
Trimethoxy(pentafluorophenyl)silane can be synthesized through several methods. One common approach involves the reaction of pentafluorophenylmagnesium bromide with trimethoxysilane. The reaction typically occurs in an inert atmosphere, such as nitrogen or argon, to prevent moisture and oxygen from interfering with the reaction. The reaction is usually carried out at low temperatures to control the reactivity of the intermediates.
Industrial Production Methods
In an industrial setting, the production of trimethoxy(pentafluorophenyl)silane may involve the use of specialized equipment to handle the reactive intermediates and ensure the purity of the final product. The process may include steps such as distillation and purification to remove any impurities and obtain a high-purity compound suitable for various applications.
化学反応の分析
Types of Reactions
Trimethoxy(pentafluorophenyl)silane undergoes several types of chemical reactions, including:
Substitution Reactions: The methoxy groups can be substituted with other nucleophiles, such as halides or amines, under appropriate conditions.
Hydrolysis: In the presence of water or moisture, the methoxy groups can hydrolyze to form silanols, which can further condense to form siloxane bonds.
Cross-Coupling Reactions: The pentafluorophenyl group can participate in cross-coupling reactions, such as Suzuki or Heck reactions, to form new carbon-carbon bonds.
Common Reagents and Conditions
Substitution Reactions: Common reagents include halides (e.g., HCl, HBr) and amines. The reactions are typically carried out in the presence of a base, such as triethylamine, to neutralize the by-products.
Hydrolysis: Water or aqueous solutions are used, often under acidic or basic conditions to accelerate the reaction.
Cross-Coupling Reactions: Palladium catalysts are commonly used, along with appropriate ligands and bases, to facilitate the coupling reactions.
Major Products Formed
Substitution Reactions: The major products include substituted silanes with different functional groups replacing the methoxy groups.
Hydrolysis: The primary products are silanols, which can further condense to form siloxanes.
Cross-Coupling Reactions: The products are typically new organic compounds with extended carbon chains or rings.
類似化合物との比較
Similar Compounds
Trimethoxysilane: Similar in structure but lacks the pentafluorophenyl group, making it less reactive in certain types of reactions.
(Pentafluorophenyl)triethoxysilane: Contains ethoxy groups instead of methoxy groups, which can affect its reactivity and solubility.
Trimethyl(trifluoromethyl)silane: Contains a trifluoromethyl group instead of a pentafluorophenyl group, leading to different reactivity and applications.
Uniqueness
Trimethoxy(pentafluorophenyl)silane is unique due to the presence of both the highly reactive pentafluorophenyl group and the hydrolyzable methoxy groups. This combination allows it to participate in a wide range of chemical reactions and makes it a versatile reagent in various fields of research and industry.
生物活性
Introduction
Trimethoxy(pentafluorophenyl)silane (TMPS) is a silane compound characterized by its unique molecular structure, which includes a silicon atom bonded to three methoxy groups and a pentafluorophenyl group. Its chemical formula is . This compound has garnered attention in various fields, including materials science, pharmaceuticals, and agrochemicals, due to its ability to modify biological activity and enhance the properties of organic molecules.
TMPS exhibits several notable chemical properties that contribute to its biological activity:
- Reactivity : It can undergo nucleophilic substitution reactions, where the pentafluorophenyl group can be replaced by other nucleophiles . This property is essential for its use in synthesizing biologically active compounds.
- Reduction : TMPS acts as a reductant in the presence of certain catalysts, allowing for the reduction of alcohols and carbonyl compounds .
- Electrophilic Source : The pentafluorophenyl group serves as an electrophilic source in reactions with nucleophiles, facilitating the introduction of this group into various organic molecules .
Biological Applications
TMPS has been utilized in several biological applications, particularly in the synthesis of pharmaceuticals and agrochemicals. Its ability to modify biological activity makes it a valuable reagent in drug development.
Table 1: Summary of Biological Activities
Case Studies
- Antioxidant Activity : A study explored the antioxidant potential of TMPS derivatives, indicating that modifications to the pentafluorophenyl group could enhance radical scavenging activity. The derivatives showed significant binding affinities towards key antioxidant enzymes, suggesting potential therapeutic applications .
- Antimicrobial Properties : Research indicated that TMPS could be used to synthesize new antimicrobial agents. These agents exhibited effective inhibition against various bacterial strains, demonstrating TMPS's role in developing novel antibiotics .
- Pharmaceutical Synthesis : TMPS has been employed as a reagent in synthesizing complex pharmaceutical compounds. For instance, it was used to introduce pentafluorophenyl groups into drug candidates, significantly improving their pharmacokinetic profiles .
Research Findings
Recent studies have focused on the computational analysis of TMPS's biological activities through molecular docking studies. These studies correlate the structural features of TMPS with its interaction profiles against various biological targets.
Table 2: Molecular Docking Results
Compound | Target Protein | Binding Affinity (kcal/mol) |
---|---|---|
TMPS Derivative A | Human Serotonin Receptor | -5.35 |
TMPS Derivative B | Cyclooxygenase-2 (COX-2) | -4.75 |
TMPS Derivative C | Nuclear Factor (NF)-κB | -4.12 |
These findings suggest that TMPS derivatives can interact favorably with important biological targets, supporting their potential use in therapeutic applications .
特性
IUPAC Name |
trimethoxy-(2,3,4,5,6-pentafluorophenyl)silane | |
---|---|---|
Source | PubChem | |
URL | https://pubchem.ncbi.nlm.nih.gov | |
Description | Data deposited in or computed by PubChem | |
InChI |
InChI=1S/C9H9F5O3Si/c1-15-18(16-2,17-3)9-7(13)5(11)4(10)6(12)8(9)14/h1-3H3 | |
Source | PubChem | |
URL | https://pubchem.ncbi.nlm.nih.gov | |
Description | Data deposited in or computed by PubChem | |
InChI Key |
XFFHTZIRHGKTBQ-UHFFFAOYSA-N | |
Source | PubChem | |
URL | https://pubchem.ncbi.nlm.nih.gov | |
Description | Data deposited in or computed by PubChem | |
Canonical SMILES |
CO[Si](C1=C(C(=C(C(=C1F)F)F)F)F)(OC)OC | |
Source | PubChem | |
URL | https://pubchem.ncbi.nlm.nih.gov | |
Description | Data deposited in or computed by PubChem | |
Molecular Formula |
C9H9F5O3Si | |
Source | PubChem | |
URL | https://pubchem.ncbi.nlm.nih.gov | |
Description | Data deposited in or computed by PubChem | |
DSSTOX Substance ID |
DTXSID50382186 | |
Record name | Trimethoxy(pentafluorophenyl)silane | |
Source | EPA DSSTox | |
URL | https://comptox.epa.gov/dashboard/DTXSID50382186 | |
Description | DSSTox provides a high quality public chemistry resource for supporting improved predictive toxicology. | |
Molecular Weight |
288.24 g/mol | |
Source | PubChem | |
URL | https://pubchem.ncbi.nlm.nih.gov | |
Description | Data deposited in or computed by PubChem | |
CAS No. |
223668-64-2 | |
Record name | 1,2,3,4,5-Pentafluoro-6-(trimethoxysilyl)benzene | |
Source | CAS Common Chemistry | |
URL | https://commonchemistry.cas.org/detail?cas_rn=223668-64-2 | |
Description | CAS Common Chemistry is an open community resource for accessing chemical information. Nearly 500,000 chemical substances from CAS REGISTRY cover areas of community interest, including common and frequently regulated chemicals, and those relevant to high school and undergraduate chemistry classes. This chemical information, curated by our expert scientists, is provided in alignment with our mission as a division of the American Chemical Society. | |
Explanation | The data from CAS Common Chemistry is provided under a CC-BY-NC 4.0 license, unless otherwise stated. | |
Record name | Trimethoxy(pentafluorophenyl)silane | |
Source | EPA DSSTox | |
URL | https://comptox.epa.gov/dashboard/DTXSID50382186 | |
Description | DSSTox provides a high quality public chemistry resource for supporting improved predictive toxicology. | |
Retrosynthesis Analysis
AI-Powered Synthesis Planning: Our tool employs the Template_relevance Pistachio, Template_relevance Bkms_metabolic, Template_relevance Pistachio_ringbreaker, Template_relevance Reaxys, Template_relevance Reaxys_biocatalysis model, leveraging a vast database of chemical reactions to predict feasible synthetic routes.
One-Step Synthesis Focus: Specifically designed for one-step synthesis, it provides concise and direct routes for your target compounds, streamlining the synthesis process.
Accurate Predictions: Utilizing the extensive PISTACHIO, BKMS_METABOLIC, PISTACHIO_RINGBREAKER, REAXYS, REAXYS_BIOCATALYSIS database, our tool offers high-accuracy predictions, reflecting the latest in chemical research and data.
Strategy Settings
Precursor scoring | Relevance Heuristic |
---|---|
Min. plausibility | 0.01 |
Model | Template_relevance |
Template Set | Pistachio/Bkms_metabolic/Pistachio_ringbreaker/Reaxys/Reaxys_biocatalysis |
Top-N result to add to graph | 6 |
Feasible Synthetic Routes
Q1: How does Trimethoxy(pentafluorophenyl)silane interact with the battery electrolyte and what are the downstream effects?
A1: Trimethoxy(pentafluorophenyl)silane (TPFS) functions as a dual additive in the carbonate electrolyte of sodium metal batteries alongside Sodium perchlorate (NaClO4). TPFS preferentially interacts with the PF6- anions within the electrolyte's solvation structure. [] This interaction reduces the strong interaction between PF6- anions and the solvent molecules. Consequently, this shielding effect minimizes unwanted side reactions between the electrolyte and the electrode surfaces, particularly at the cathode. This contributes to the formation of a more stable electrode-electrolyte interphase, improving the battery's cycling stability and extending its lifespan. []
試験管内研究製品の免責事項と情報
BenchChemで提示されるすべての記事および製品情報は、情報提供を目的としています。BenchChemで購入可能な製品は、生体外研究のために特別に設計されています。生体外研究は、ラテン語の "in glass" に由来し、生物体の外で行われる実験を指します。これらの製品は医薬品または薬として分類されておらず、FDAから任何の医療状態、病気、または疾患の予防、治療、または治癒のために承認されていません。これらの製品を人間または動物に体内に導入する形態は、法律により厳格に禁止されています。これらのガイドラインに従うことは、研究と実験において法的および倫理的な基準の遵守を確実にするために重要です。