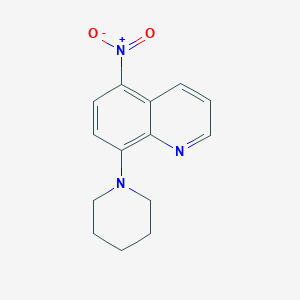
5-Nitro-8-(piperidin-1-yl)quinoline
- 専門家チームからの見積もりを受け取るには、QUICK INQUIRYをクリックしてください。
- 品質商品を競争力のある価格で提供し、研究に集中できます。
説明
5-Nitro-8-(piperidin-1-yl)quinoline is a chemical compound with the molecular formula C14H15N3O2 . It has a molecular weight of 257.29 . The compound is used for research purposes .
Molecular Structure Analysis
The InChI code for 5-Nitro-8-(piperidin-1-yl)quinoline is1S/C14H15N3O2/c18-17(19)12-6-7-13(16-9-2-1-3-10-16)14-11(12)5-4-8-15-14/h4-8H,1-3,9-10H2
. This code provides a specific description of the molecule’s structure. Physical And Chemical Properties Analysis
The physical and chemical properties of 5-Nitro-8-(piperidin-1-yl)quinoline include a density of 1.3±0.1 g/cm³ . The boiling point is 461.7±35.0 °C at 760 mmHg . The compound has a molar refractivity of 73.5±0.3 cm³ . It has 5 H bond acceptors, 0 H bond donors, and 2 freely rotating bonds .科学的研究の応用
Pharmaceutical Industry
Piperidine derivatives, such as 5-Nitro-8-(piperidin-1-yl)quinoline, play a significant role in the pharmaceutical industry . They are present in more than twenty classes of pharmaceuticals . The pharmaceutical applications of synthetic and natural piperidines were covered, as well as the latest scientific advances in the discovery and biological evaluation of potential drugs containing piperidine moiety .
Anticancer Agents
Piperidine derivatives are being utilized in different ways as anticancer agents . The specific role of 5-Nitro-8-(piperidin-1-yl)quinoline in this field could be a subject of further research.
Antiviral Agents
Piperidine derivatives are also used as antiviral agents . The specific role of 5-Nitro-8-(piperidin-1-yl)quinoline in this field could be a subject of further research.
Antimalarial Agents
Piperidine derivatives are used as antimalarial agents . The specific role of 5-Nitro-8-(piperidin-1-yl)quinoline in this field could be a subject of further research.
Antimicrobial and Antifungal Agents
Piperidine derivatives are used as antimicrobial and antifungal agents . The specific role of 5-Nitro-8-(piperidin-1-yl)quinoline in this field could be a subject of further research.
Antihypertension, Analgesic, and Anti-inflammatory Agents
Piperidine derivatives are used as antihypertension, analgesic, and anti-inflammatory agents . The specific role of 5-Nitro-8-(piperidin-1-yl)quinoline in these fields could be a subject of further research.
Anti-Alzheimer Agents
Piperidine derivatives are used as anti-Alzheimer agents . The specific role of 5-Nitro-8-(piperidin-1-yl)quinoline in this field could be a subject of further research.
Antipsychotic and/or Anticoagulant Agents
Piperidine derivatives are used as antipsychotic and/or anticoagulant agents . The specific role of 5-Nitro-8-(piperidin-1-yl)quinoline in these fields could be a subject of further research.
Safety and Hazards
The safety data sheet (SDS) for 5-Nitro-8-(piperidin-1-yl)quinoline indicates that it is harmful by inhalation, in contact with skin, and if swallowed . In case of skin contact, it is recommended to wash the skin with copious amounts of water for at least 15 minutes while removing contaminated clothing and shoes .
作用機序
Mode of Action
It is known that the piperidine nucleus plays a crucial role in the inhibitory effect of similar compounds
Pharmacokinetics
The compound’s molecular weight of 257.3 suggests it may have suitable properties for absorption and distribution, but further studies are required to confirm this.
Action Environment
Environmental factors can significantly influence the action, efficacy, and stability of a compound. Factors such as temperature, pH, and the presence of other molecules can affect how 5-Nitro-8-(piperidin-1-yl)quinoline interacts with its targets. For instance, the compound is recommended to be stored at 2-8°C , suggesting that temperature could influence its stability.
特性
IUPAC Name |
5-nitro-8-piperidin-1-ylquinoline |
Source
|
---|---|---|
Source | PubChem | |
URL | https://pubchem.ncbi.nlm.nih.gov | |
Description | Data deposited in or computed by PubChem | |
InChI |
InChI=1S/C14H15N3O2/c18-17(19)12-6-7-13(16-9-2-1-3-10-16)14-11(12)5-4-8-15-14/h4-8H,1-3,9-10H2 |
Source
|
Source | PubChem | |
URL | https://pubchem.ncbi.nlm.nih.gov | |
Description | Data deposited in or computed by PubChem | |
InChI Key |
MJKNJLAZNNSLRM-UHFFFAOYSA-N |
Source
|
Source | PubChem | |
URL | https://pubchem.ncbi.nlm.nih.gov | |
Description | Data deposited in or computed by PubChem | |
Canonical SMILES |
C1CCN(CC1)C2=C3C(=C(C=C2)[N+](=O)[O-])C=CC=N3 |
Source
|
Source | PubChem | |
URL | https://pubchem.ncbi.nlm.nih.gov | |
Description | Data deposited in or computed by PubChem | |
Molecular Formula |
C14H15N3O2 |
Source
|
Source | PubChem | |
URL | https://pubchem.ncbi.nlm.nih.gov | |
Description | Data deposited in or computed by PubChem | |
DSSTOX Substance ID |
DTXSID60389525 |
Source
|
Record name | 5-nitro-8-(piperidin-1-yl)quinoline | |
Source | EPA DSSTox | |
URL | https://comptox.epa.gov/dashboard/DTXSID60389525 | |
Description | DSSTox provides a high quality public chemistry resource for supporting improved predictive toxicology. | |
Molecular Weight |
257.29 g/mol |
Source
|
Source | PubChem | |
URL | https://pubchem.ncbi.nlm.nih.gov | |
Description | Data deposited in or computed by PubChem | |
Product Name |
5-Nitro-8-(piperidin-1-yl)quinoline | |
CAS RN |
142315-99-9 |
Source
|
Record name | 5-nitro-8-(piperidin-1-yl)quinoline | |
Source | EPA DSSTox | |
URL | https://comptox.epa.gov/dashboard/DTXSID60389525 | |
Description | DSSTox provides a high quality public chemistry resource for supporting improved predictive toxicology. | |
試験管内研究製品の免責事項と情報
BenchChemで提示されるすべての記事および製品情報は、情報提供を目的としています。BenchChemで購入可能な製品は、生体外研究のために特別に設計されています。生体外研究は、ラテン語の "in glass" に由来し、生物体の外で行われる実験を指します。これらの製品は医薬品または薬として分類されておらず、FDAから任何の医療状態、病気、または疾患の予防、治療、または治癒のために承認されていません。これらの製品を人間または動物に体内に導入する形態は、法律により厳格に禁止されています。これらのガイドラインに従うことは、研究と実験において法的および倫理的な基準の遵守を確実にするために重要です。