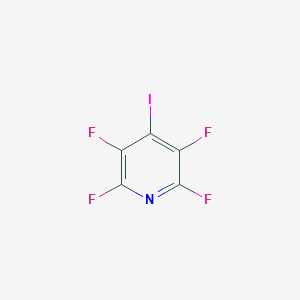
2,3,5,6-Tetrafluoro-4-iodopyridine
概要
説明
2,3,5,6-Tetrafluoro-4-iodopyridine is a fluorinated heterocyclic compound It is a derivative of pyridine, where four hydrogen atoms are replaced by fluorine atoms and one hydrogen atom is replaced by an iodine atom
科学的研究の応用
2,3,5,6-Tetrafluoro-4-iodopyridine has several applications in scientific research. It is used in the synthesis of fluorinated pyridines, which are valuable in medicinal chemistry due to their biological activity . The compound is also used in the development of imaging agents for biological applications .
Safety and Hazards
作用機序
Target of Action
It’s known that fluorinated pyridines are generally used as molecular scaffolds for active pharmaceutical ingredients (apis) and substrates for synthetic chemistry .
Mode of Action
The mode of action of 2,3,5,6-Tetrafluoro-4-iodopyridine involves its interaction with various ions. Hydroxide ion, methoxide ion, and ammonia attack this compound at the 2-position, giving the corresponding 2-substituted trifluoro-4-iodopyridines . Iodide ion appears to attack the 4-iodo-substituent, resulting in the formation of the 2,3,5,6-tetrafluoropyridyl anion .
Biochemical Pathways
Fluorinated pyridines are known to have interesting and unusual physical, chemical, and biological properties due to the presence of strong electron-withdrawing substituents in the aromatic ring .
Pharmacokinetics
The presence of fluorine atoms in the compound could potentially influence its bioavailability, as fluorine atoms are often introduced into lead structures in the search for new pharmaceuticals with improved physical, biological, and environmental properties .
Result of Action
Fluorinated pyridines are known to be less reactive than their chlorinated and brominated analogues due to their reduced basicity .
Action Environment
The presence of fluorine atoms in the compound could potentially influence its stability and reactivity in different environments .
準備方法
2,3,5,6-Tetrafluoro-4-iodopyridine can be synthesized through several methods. One common method involves the oxidation of 2,3,5,6-tetrafluoro-4-hydrazinopyridine in the presence of methyl iodide. Another method involves the reaction of pentafluoropyridine with sodium iodide in dimethylformamide . These methods yield the desired compound with high efficiency and purity.
化学反応の分析
2,3,5,6-Tetrafluoro-4-iodopyridine undergoes various chemical reactions, including nucleophilic substitution and coupling reactions. For instance, hydroxide ion, methoxide ion, and ammonia can attack the compound at the 2-position, resulting in the formation of 2-substituted trifluoro-4-iodopyridines . Additionally, the compound can be converted into 2,3,5,6-tetrafluoropyridylmagnesium iodide or 2,3,5,6-tetrafluoropyridyllithium, which are useful intermediates in organic synthesis .
類似化合物との比較
2,3,5,6-Tetrafluoro-4-iodopyridine is unique compared to other fluorinated pyridines due to the presence of the iodine atom. Similar compounds include 2,3,5,6-tetrafluoropyridine and 3-chloro-2,4,5,6-tetrafluoropyridine . These compounds share similar fluorination patterns but differ in their reactivity and applications. The iodine atom in this compound provides unique reactivity, making it a valuable intermediate in organic synthesis.
特性
IUPAC Name |
2,3,5,6-tetrafluoro-4-iodopyridine | |
---|---|---|
Source | PubChem | |
URL | https://pubchem.ncbi.nlm.nih.gov | |
Description | Data deposited in or computed by PubChem | |
InChI |
InChI=1S/C5F4IN/c6-1-3(10)2(7)5(9)11-4(1)8 | |
Source | PubChem | |
URL | https://pubchem.ncbi.nlm.nih.gov | |
Description | Data deposited in or computed by PubChem | |
InChI Key |
HNPRQTRSDKIVFY-UHFFFAOYSA-N | |
Source | PubChem | |
URL | https://pubchem.ncbi.nlm.nih.gov | |
Description | Data deposited in or computed by PubChem | |
Canonical SMILES |
C1(=C(C(=C(N=C1F)F)F)I)F | |
Source | PubChem | |
URL | https://pubchem.ncbi.nlm.nih.gov | |
Description | Data deposited in or computed by PubChem | |
Molecular Formula |
C5F4IN | |
Source | PubChem | |
URL | https://pubchem.ncbi.nlm.nih.gov | |
Description | Data deposited in or computed by PubChem | |
Molecular Weight |
276.96 g/mol | |
Source | PubChem | |
URL | https://pubchem.ncbi.nlm.nih.gov | |
Description | Data deposited in or computed by PubChem | |
Retrosynthesis Analysis
AI-Powered Synthesis Planning: Our tool employs the Template_relevance Pistachio, Template_relevance Bkms_metabolic, Template_relevance Pistachio_ringbreaker, Template_relevance Reaxys, Template_relevance Reaxys_biocatalysis model, leveraging a vast database of chemical reactions to predict feasible synthetic routes.
One-Step Synthesis Focus: Specifically designed for one-step synthesis, it provides concise and direct routes for your target compounds, streamlining the synthesis process.
Accurate Predictions: Utilizing the extensive PISTACHIO, BKMS_METABOLIC, PISTACHIO_RINGBREAKER, REAXYS, REAXYS_BIOCATALYSIS database, our tool offers high-accuracy predictions, reflecting the latest in chemical research and data.
Strategy Settings
Precursor scoring | Relevance Heuristic |
---|---|
Min. plausibility | 0.01 |
Model | Template_relevance |
Template Set | Pistachio/Bkms_metabolic/Pistachio_ringbreaker/Reaxys/Reaxys_biocatalysis |
Top-N result to add to graph | 6 |
Feasible Synthetic Routes
Q1: What are the key reactions of 2,3,5,6-tetrafluoro-4-iodopyridine highlighted in the research?
A1: The research primarily focuses on utilizing this compound as a starting material for further synthesis. Key reactions include:
- Formation of Organometallic Reagents: The iodine atom can be readily replaced with magnesium or lithium, forming 2,3,5,6-tetrafluoropyridylmagnesium iodide or 2,3,5,6-tetrafluoropyridyllithium, respectively. These organometallic compounds are valuable intermediates in organic synthesis. []
- Nucleophilic Substitution: Nucleophiles like hydroxide, methoxide, and ammonia preferentially attack the 2-position of the pyridine ring, displacing a fluorine atom and creating 2-substituted trifluoro-4-iodopyridines. []
- Coupling Reactions: this compound can undergo Ullmann coupling, leading to the formation of perfluoro-4,4′-bipyridyl. This reaction demonstrates its potential in constructing complex fluorinated nitrogen-containing heterocycles. []
Q2: Why is the synthesis of this compound significant?
A2: This compound serves as a crucial building block for synthesizing various fluorinated pyridines. The presence of both iodine and fluorine allows for diverse reactivity, enabling the introduction of different substituents and the creation of complex molecules. This is particularly relevant for developing new materials and pharmaceuticals, where fluorinated compounds are gaining increasing importance. []
試験管内研究製品の免責事項と情報
BenchChemで提示されるすべての記事および製品情報は、情報提供を目的としています。BenchChemで購入可能な製品は、生体外研究のために特別に設計されています。生体外研究は、ラテン語の "in glass" に由来し、生物体の外で行われる実験を指します。これらの製品は医薬品または薬として分類されておらず、FDAから任何の医療状態、病気、または疾患の予防、治療、または治癒のために承認されていません。これらの製品を人間または動物に体内に導入する形態は、法律により厳格に禁止されています。これらのガイドラインに従うことは、研究と実験において法的および倫理的な基準の遵守を確実にするために重要です。