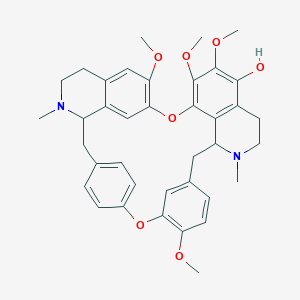
Thalidezine
概要
説明
Thalidezine is a bisbenzylisoquinoline alkaloid known for its role as a novel activator of AMP-activated protein kinase (AMPK). This compound has garnered attention due to its ability to induce autophagic cell death in apoptosis-resistant cancer cells, making it a promising candidate for cancer therapy .
準備方法
Synthetic Routes and Reaction Conditions: Thalidezine can be synthesized through a series of organic reactions involving the coupling of specific isoquinoline derivatives. The exact synthetic route may vary, but it typically involves the formation of the bisbenzylisoquinoline core structure followed by functional group modifications to achieve the desired compound .
Industrial Production Methods: Industrial production of this compound would likely involve optimizing the synthetic route for large-scale production, ensuring high yield and purity. This may include the use of advanced catalytic processes and purification techniques to isolate this compound from reaction mixtures .
化学反応の分析
Types of Reactions: Thalidezine undergoes various chemical reactions, including:
Oxidation: this compound can be oxidized to form different derivatives, which may exhibit distinct biological activities.
Reduction: Reduction reactions can modify the functional groups on this compound, potentially altering its pharmacological properties.
Common Reagents and Conditions:
Oxidation: Common oxidizing agents such as potassium permanganate or chromium trioxide may be used.
Reduction: Reducing agents like sodium borohydride or lithium aluminum hydride are typically employed.
Substitution: Various nucleophiles or electrophiles can be used under appropriate conditions to achieve substitution reactions.
Major Products Formed: The major products formed from these reactions depend on the specific reagents and conditions used. For example, oxidation may yield quinone derivatives, while reduction could produce dihydro derivatives .
科学的研究の応用
Thalidezine has several scientific research applications, including:
Chemistry: this compound serves as a valuable compound for studying the mechanisms of AMPK activation and autophagy.
Biology: It is used to investigate cellular energy homeostasis and metabolic regulation.
Medicine: this compound shows potential in cancer therapy, particularly for treating apoptosis-resistant cancers by inducing autophagic cell death
Industry: The antimicrobial properties of this compound make it useful in developing new antimicrobial agents.
作用機序
Thalidezine exerts its effects primarily through the activation of AMP-activated protein kinase (AMPK). AMPK is a central regulator of cellular energy homeostasis, and its activation leads to various downstream effects, including the induction of autophagy. This compound directly activates AMPK, which in turn increases autophagic flux in cancer cells, leading to autophagic cell death. This mechanism is particularly effective in overcoming apoptosis resistance in cancer cells .
類似化合物との比較
Berberine: Another bisbenzylisoquinoline alkaloid with AMPK-activating properties.
Palmatine: Similar in structure and function, also known for its antimicrobial and anticancer activities.
Tetrandrine: A bisbenzylisoquinoline alkaloid with anti-inflammatory and anticancer properties
Uniqueness of Thalidezine: this compound is unique due to its potent and direct activation of AMPK, leading to significant autophagic cell death in apoptosis-resistant cancer cells. This makes it a promising candidate for developing new cancer therapies, particularly for cancers that are resistant to conventional treatments .
生物活性
Thalidomide, originally developed as a sedative in the late 1950s, is now recognized for its complex biological activity, particularly in treating various medical conditions, including multiple myeloma and leprosy. This article aims to provide an in-depth analysis of thalidomide's biological activity, highlighting its pharmacokinetics, mechanisms of action, therapeutic applications, and notable case studies.
Pharmacokinetics and Metabolism
Thalidomide exhibits significant interspecies differences in pharmacokinetics, which can influence its biological effects. A study conducted on mice and rabbits showed that after administration of thalidomide at a dose of 2 mg/kg, the area under the concentration curve (AUC) values were markedly different among species: mice had an AUC of 4 micromol/L·hour, rabbits 8 micromol/L·hour, and multiple myeloma patients 81 micromol/L·hour. The elimination half-lives also varied significantly: 0.5 hours in mice, 2.2 hours in rabbits, and 7.3 hours in patients .
Table 1: Pharmacokinetic Parameters of Thalidomide Across Species
Species | AUC (µmol/L·hour) | Elimination Half-Life (hours) |
---|---|---|
Mice | 4 | 0.5 |
Rabbits | 8 | 2.2 |
Multiple Myeloma Patients | 81 | 7.3 |
These differences in metabolism may account for the varying biological effects observed across species.
Thalidomide's mechanisms of action are multifaceted:
- Immune Modulation : Thalidomide acts as an immunomodulatory drug (IMiD), inhibiting tumor necrosis factor (TNF) secretion, which plays a crucial role in inflammatory responses .
- Anti-Angiogenesis : It inhibits angiogenesis by targeting specific pathways involved in blood vessel formation, aiding in the treatment of cancers such as multiple myeloma .
- Cytokine Regulation : Thalidomide influences cytokine production, particularly enhancing the production of interleukin-10 (IL-10), which has anti-inflammatory properties .
Therapeutic Applications
Thalidomide has been approved for several medical uses:
- Multiple Myeloma : Thalidomide is used as part of combination therapy for multiple myeloma, showing response rates exceeding 60% when combined with dexamethasone . Its efficacy has led to extended survival rates for patients.
- Erythema Nodosum Leprosum : The drug is also approved for treating this painful skin condition associated with leprosy .
- COVID-19 : Recent studies suggest thalidomide may reduce inflammation in COVID-19 patients when used alongside glucocorticoids. In a small clinical trial, thalidomide was shown to accelerate viral clearance and reduce hospital stays .
Case Studies
- Thalidomide Embryopathy : A notable case involved a woman whose mother took thalidomide during pregnancy. The patient exhibited multiple physical deformities consistent with thalidomide embryopathy, highlighting the drug's teratogenic effects when used during gestation .
- Thalidomide in COVID-19 Treatment : In a case study involving six patients with severe COVID-19 symptoms, those treated with thalidomide alongside low-dose dexamethasone showed improved outcomes compared to controls. The treatment was associated with reduced inflammatory cytokines and shorter hospital stays .
特性
IUPAC Name |
9,20,21,25-tetramethoxy-15,30-dimethyl-7,23-dioxa-15,30-diazaheptacyclo[22.6.2.23,6.18,12.114,18.027,31.022,33]hexatriaconta-3(36),4,6(35),8,10,12(34),18(33),19,21,24,26,31-dodecaen-19-ol | |
---|---|---|
Source | PubChem | |
URL | https://pubchem.ncbi.nlm.nih.gov | |
Description | Data deposited in or computed by PubChem | |
InChI |
InChI=1S/C38H42N2O7/c1-39-15-13-24-20-31(43-4)33-21-27(24)28(39)17-22-7-10-25(11-8-22)46-32-19-23(9-12-30(32)42-3)18-29-34-26(14-16-40(29)2)35(41)37(44-5)38(45-6)36(34)47-33/h7-12,19-21,28-29,41H,13-18H2,1-6H3 | |
Source | PubChem | |
URL | https://pubchem.ncbi.nlm.nih.gov | |
Description | Data deposited in or computed by PubChem | |
InChI Key |
UYNHKOIUEXICNQ-UHFFFAOYSA-N | |
Source | PubChem | |
URL | https://pubchem.ncbi.nlm.nih.gov | |
Description | Data deposited in or computed by PubChem | |
Canonical SMILES |
CN1CCC2=CC(=C3C=C2C1CC4=CC=C(C=C4)OC5=C(C=CC(=C5)CC6C7=C(CCN6C)C(=C(C(=C7O3)OC)OC)O)OC)OC | |
Source | PubChem | |
URL | https://pubchem.ncbi.nlm.nih.gov | |
Description | Data deposited in or computed by PubChem | |
Molecular Formula |
C38H42N2O7 | |
Source | PubChem | |
URL | https://pubchem.ncbi.nlm.nih.gov | |
Description | Data deposited in or computed by PubChem | |
DSSTOX Substance ID |
DTXSID20313346 | |
Record name | THALIDEZINE | |
Source | EPA DSSTox | |
URL | https://comptox.epa.gov/dashboard/DTXSID20313346 | |
Description | DSSTox provides a high quality public chemistry resource for supporting improved predictive toxicology. | |
Molecular Weight |
638.7 g/mol | |
Source | PubChem | |
URL | https://pubchem.ncbi.nlm.nih.gov | |
Description | Data deposited in or computed by PubChem | |
CAS No. |
18251-36-0 | |
Record name | THALIDEZINE | |
Source | DTP/NCI | |
URL | https://dtp.cancer.gov/dtpstandard/servlet/dwindex?searchtype=NSC&outputformat=html&searchlist=269188 | |
Description | The NCI Development Therapeutics Program (DTP) provides services and resources to the academic and private-sector research communities worldwide to facilitate the discovery and development of new cancer therapeutic agents. | |
Explanation | Unless otherwise indicated, all text within NCI products is free of copyright and may be reused without our permission. Credit the National Cancer Institute as the source. | |
Record name | THALIDEZINE | |
Source | EPA DSSTox | |
URL | https://comptox.epa.gov/dashboard/DTXSID20313346 | |
Description | DSSTox provides a high quality public chemistry resource for supporting improved predictive toxicology. | |
Retrosynthesis Analysis
AI-Powered Synthesis Planning: Our tool employs the Template_relevance Pistachio, Template_relevance Bkms_metabolic, Template_relevance Pistachio_ringbreaker, Template_relevance Reaxys, Template_relevance Reaxys_biocatalysis model, leveraging a vast database of chemical reactions to predict feasible synthetic routes.
One-Step Synthesis Focus: Specifically designed for one-step synthesis, it provides concise and direct routes for your target compounds, streamlining the synthesis process.
Accurate Predictions: Utilizing the extensive PISTACHIO, BKMS_METABOLIC, PISTACHIO_RINGBREAKER, REAXYS, REAXYS_BIOCATALYSIS database, our tool offers high-accuracy predictions, reflecting the latest in chemical research and data.
Strategy Settings
Precursor scoring | Relevance Heuristic |
---|---|
Min. plausibility | 0.01 |
Model | Template_relevance |
Template Set | Pistachio/Bkms_metabolic/Pistachio_ringbreaker/Reaxys/Reaxys_biocatalysis |
Top-N result to add to graph | 6 |
Feasible Synthetic Routes
Q1: What is the mechanism of action of thalidezine?
A1: this compound is a bisbenzylisoquinoline alkaloid isolated from plants of the Thalictrum genus [, , ]. It acts as a direct activator of AMP-activated protein kinase (AMPK) []. By directly binding to AMPK, this compound promotes its activation, leading to downstream effects on cellular energy homeostasis, metabolism, and autophagy [].
Q2: How does this compound impact cancer cells?
A2: this compound demonstrates promising anticancer activity, particularly against apoptosis-resistant cancer cells []. Instead of inducing apoptosis (programmed cell death), this compound triggers energy-mediated autophagic cell death in these resistant cancer cells []. This effect is dependent on AMPK activation, as demonstrated by the ability of AMPK inhibitors to abolish this compound-induced cell death [].
Q3: What is the significance of this compound's ability to induce autophagic cell death in apoptosis-resistant cancer cells?
A3: Resistance to apoptosis is a major hurdle in cancer treatment, often rendering conventional chemotherapy ineffective. This compound's ability to bypass this resistance mechanism by inducing an alternative cell death pathway (autophagy) makes it a promising candidate for further development as a chemotherapeutic agent, especially for cancers exhibiting multidrug resistance [].
Q4: Beyond its anticancer activity, what other biological activities have been reported for this compound?
A4: Research on this compound and its analogs, such as hernandezine and isothis compound, also extracted from Thalictrum species, reveals inhibitory effects on the growth of various cancer cell lines, including P388 leukemia, S180 ascites, C26 colon cancer, mouse L1210 cells, and human oral cancer KB cells []. Notably, hernandezine exhibits a lower inhibitory effect on normal hematopoietic progenitor cells compared to cancerous cells, suggesting a potential therapeutic window [].
Q5: Where can I find detailed information about the isolation and structural characterization of this compound?
A5: The isolation and characterization of this compound, along with other alkaloids, from Thalictrum longistylum was first reported in a study by Shamma et al. []. This study provides insights into the structure elucidation of this compound, utilizing spectral and chemical methods, including NMR studies and chemical conversions [].
試験管内研究製品の免責事項と情報
BenchChemで提示されるすべての記事および製品情報は、情報提供を目的としています。BenchChemで購入可能な製品は、生体外研究のために特別に設計されています。生体外研究は、ラテン語の "in glass" に由来し、生物体の外で行われる実験を指します。これらの製品は医薬品または薬として分類されておらず、FDAから任何の医療状態、病気、または疾患の予防、治療、または治癒のために承認されていません。これらの製品を人間または動物に体内に導入する形態は、法律により厳格に禁止されています。これらのガイドラインに従うことは、研究と実験において法的および倫理的な基準の遵守を確実にするために重要です。