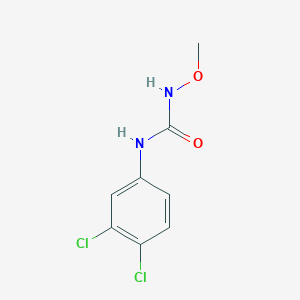
N-(3,4-Dichlorophenyl)-N'-methoxyurea
説明
“N-(3,4-Dichlorophenyl)-N’-methoxyurea” is a chemical compound that likely contains a urea group (-NH-CO-NH-) attached to a 3,4-dichlorophenyl group and a methoxy group. Urea derivatives are widely used in medicinal chemistry and agriculture .
Molecular Structure Analysis
The molecular structure of “N-(3,4-Dichlorophenyl)-N’-methoxyurea” would likely show the presence of a urea functional group attached to a 3,4-dichlorophenyl group and a methoxy group . The exact structure and conformation would depend on the specific conditions and environment .Chemical Reactions Analysis
Urea derivatives can participate in a variety of chemical reactions, including hydrolysis, condensation, and addition reactions . The specific reactions that “N-(3,4-Dichlorophenyl)-N’-methoxyurea” might undergo would depend on the reaction conditions and the presence of other reactants .Physical And Chemical Properties Analysis
The physical and chemical properties of “N-(3,4-Dichlorophenyl)-N’-methoxyurea” would depend on its specific structure. Urea derivatives generally have moderate polarity and can form hydrogen bonds, which can affect their solubility, melting point, and other properties .科学的研究の応用
Absorption and Metabolism in Plants
Research has shown that N-(3,4-Dichlorophenyl)-N'-methoxyurea, also known as linuron, is absorbed and metabolized by various plants. In a study on corn, soybean, and crabgrass, linuron was found to enter the plants along with water absorbed from the nutrient solution. A small portion of linuron was detected in the tissue in its original form and as 3,4-dichloroaniline, indicating the process of metabolism and transformation within the plants (Nashed & Ilnicki, 1970).
Degradation by Microorganisms
Linuron induces the formation of enzymes in certain microorganisms, which are responsible for the degradation of a variety of herbicides and fungicides. This was demonstrated in a study involving Bacillus sphaericus, where linuron-induced acylamidase enzyme showed the capability to degrade different types of herbicides and fungicides (Engelhardt, Wallnöfer, & Plapp, 1971).
Environmental Impact and Remediation
Linuron's environmental impact, particularly its presence in surface drainage water, has been studied. It was found that the maximum seasonal losses of linuron in runoff were relatively low, suggesting that with proper use, the herbicide poses a minimal threat to adjacent aquatic areas (Willis, Rogers, & Southwick, 1975). Additionally, the development of methods to enhance the mineralization and degradation of linuron in contaminated environments, such as the use of cyclodextrin-based bioremediation technologies, has been researched (Villaverde et al., 2012).
Detection and Measurement Methods
Various methods have been developed for detecting and measuring linuron in environmental samples. For instance, a chromatographic procedure for determining linuron in soils was established, highlighting the degradation process of the herbicide in the soil environment (Katz & Strusz, 1968). Another study focused on the voltammetric determination of linuron using a carbon paste electrode, offering an alternative method for analyzing linuron in environmental samples (Ðordevic et al., 2011).
Photocatalytic Degradation
The photocatalytic degradation of linuron using TiO2 suspensions has been investigated as a means of treating water contaminated with the herbicide. This approach was found to be effective in degrading linuron and potentially aiding in its mineralization (Ruggieri et al., 2011).
将来の方向性
The future directions for the study of “N-(3,4-Dichlorophenyl)-N’-methoxyurea” would likely depend on its specific properties and potential applications. Urea derivatives are a rich field of study in medicinal chemistry and agriculture, and new compounds are continually being synthesized and studied .
作用機序
- As a result, the normal electron transport chain is disrupted, reducing the plant’s ability to convert light energy into chemical energy (ATP and reductant potential) .
- Notably, Linuron does not affect photosystem I or other reactions in photosynthesis, such as light absorption or carbon fixation in the Calvin cycle .
- Impact on Bioavailability : Linuron’s persistence in soil affects its availability for uptake by plants .
Target of Action
Mode of Action
Biochemical Pathways
Pharmacokinetics
Result of Action
Action Environment
特性
IUPAC Name |
1-(3,4-dichlorophenyl)-3-methoxyurea | |
---|---|---|
Source | PubChem | |
URL | https://pubchem.ncbi.nlm.nih.gov | |
Description | Data deposited in or computed by PubChem | |
InChI |
InChI=1S/C8H8Cl2N2O2/c1-14-12-8(13)11-5-2-3-6(9)7(10)4-5/h2-4H,1H3,(H2,11,12,13) | |
Source | PubChem | |
URL | https://pubchem.ncbi.nlm.nih.gov | |
Description | Data deposited in or computed by PubChem | |
InChI Key |
QDSJDNUSIBDVMS-UHFFFAOYSA-N | |
Source | PubChem | |
URL | https://pubchem.ncbi.nlm.nih.gov | |
Description | Data deposited in or computed by PubChem | |
Canonical SMILES |
CONC(=O)NC1=CC(=C(C=C1)Cl)Cl | |
Source | PubChem | |
URL | https://pubchem.ncbi.nlm.nih.gov | |
Description | Data deposited in or computed by PubChem | |
Molecular Formula |
C8H8Cl2N2O2 | |
Source | PubChem | |
URL | https://pubchem.ncbi.nlm.nih.gov | |
Description | Data deposited in or computed by PubChem | |
DSSTOX Substance ID |
DTXSID70864772 | |
Record name | N-(3,4-Dichlorophenyl)-N'-methoxyurea | |
Source | EPA DSSTox | |
URL | https://comptox.epa.gov/dashboard/DTXSID70864772 | |
Description | DSSTox provides a high quality public chemistry resource for supporting improved predictive toxicology. | |
Molecular Weight |
235.06 g/mol | |
Source | PubChem | |
URL | https://pubchem.ncbi.nlm.nih.gov | |
Description | Data deposited in or computed by PubChem | |
CAS RN |
17356-61-5 | |
Record name | N-(3,4-Dichlorophenyl)-N'-methoxyurea | |
Source | ChemIDplus | |
URL | https://pubchem.ncbi.nlm.nih.gov/substance/?source=chemidplus&sourceid=0017356615 | |
Description | ChemIDplus is a free, web search system that provides access to the structure and nomenclature authority files used for the identification of chemical substances cited in National Library of Medicine (NLM) databases, including the TOXNET system. | |
Record name | N-(3,4-Dichlorophenyl)-N'-methoxyurea | |
Source | EPA DSSTox | |
URL | https://comptox.epa.gov/dashboard/DTXSID70864772 | |
Description | DSSTox provides a high quality public chemistry resource for supporting improved predictive toxicology. | |
Record name | N-(3,4-DICHLOROPHENYL)-N'-METHOXYUREA | |
Source | FDA Global Substance Registration System (GSRS) | |
URL | https://gsrs.ncats.nih.gov/ginas/app/beta/substances/R12X6ONT89 | |
Description | The FDA Global Substance Registration System (GSRS) enables the efficient and accurate exchange of information on what substances are in regulated products. Instead of relying on names, which vary across regulatory domains, countries, and regions, the GSRS knowledge base makes it possible for substances to be defined by standardized, scientific descriptions. | |
Explanation | Unless otherwise noted, the contents of the FDA website (www.fda.gov), both text and graphics, are not copyrighted. They are in the public domain and may be republished, reprinted and otherwise used freely by anyone without the need to obtain permission from FDA. Credit to the U.S. Food and Drug Administration as the source is appreciated but not required. | |
Retrosynthesis Analysis
AI-Powered Synthesis Planning: Our tool employs the Template_relevance Pistachio, Template_relevance Bkms_metabolic, Template_relevance Pistachio_ringbreaker, Template_relevance Reaxys, Template_relevance Reaxys_biocatalysis model, leveraging a vast database of chemical reactions to predict feasible synthetic routes.
One-Step Synthesis Focus: Specifically designed for one-step synthesis, it provides concise and direct routes for your target compounds, streamlining the synthesis process.
Accurate Predictions: Utilizing the extensive PISTACHIO, BKMS_METABOLIC, PISTACHIO_RINGBREAKER, REAXYS, REAXYS_BIOCATALYSIS database, our tool offers high-accuracy predictions, reflecting the latest in chemical research and data.
Strategy Settings
Precursor scoring | Relevance Heuristic |
---|---|
Min. plausibility | 0.01 |
Model | Template_relevance |
Template Set | Pistachio/Bkms_metabolic/Pistachio_ringbreaker/Reaxys/Reaxys_biocatalysis |
Top-N result to add to graph | 6 |
Feasible Synthetic Routes
Q & A
Q1: What are the primary metabolic pathways of linuron in rabbits, and how does this relate to N-(3,4-Dichlorophenyl)-N'-methoxyurea?
A1: Research indicates that linuron is rapidly metabolized in rabbits, primarily in the liver []. N-(3,4-Dichlorophenyl)-N'-methoxyurea is identified as a major metabolite of linuron in rabbits, both in serum samples and in vitro studies using microsomal fractions []. This suggests that N-demethylation is a key metabolic pathway for linuron in rabbits, leading to the formation of this specific metabolite.
Q2: Could the presence of linuron metabolites in rabbit feed pose any potential risks?
A2: While linuron exhibits low acute toxicity in rabbits, the study highlights a potential concern []. Linuron is known to induce liver enzymes in rats, and similar enzyme induction in rabbits exposed to linuron through contaminated feed could impact drug metabolism. This altered enzyme activity might affect the effectiveness of various veterinary drugs metabolized by the same liver enzymes, posing a risk to treated animals [].
試験管内研究製品の免責事項と情報
BenchChemで提示されるすべての記事および製品情報は、情報提供を目的としています。BenchChemで購入可能な製品は、生体外研究のために特別に設計されています。生体外研究は、ラテン語の "in glass" に由来し、生物体の外で行われる実験を指します。これらの製品は医薬品または薬として分類されておらず、FDAから任何の医療状態、病気、または疾患の予防、治療、または治癒のために承認されていません。これらの製品を人間または動物に体内に導入する形態は、法律により厳格に禁止されています。これらのガイドラインに従うことは、研究と実験において法的および倫理的な基準の遵守を確実にするために重要です。