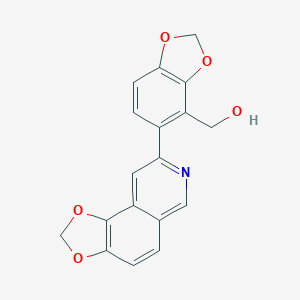
Decumbenine B
概要
説明
Decumbenine B is a naturally occurring isoquinoline alkaloid isolated from the roots of Corydalis decumbens. It has garnered significant interest due to its potential therapeutic applications, particularly in the treatment of hypertension, rheumatoid arthritis, and sciatic neuralgia .
準備方法
Synthetic Routes and Reaction Conditions
The total synthesis of Decumbenine B has been achieved through various methods. One notable method involves the use of ruthenium (III)-catalyzed direct ortho-hydroxymethylation of intermediate compounds. This method allows for the efficient synthesis of this compound from commercially available materials in just five steps, with an overall yield of 26.1% .
Another method involves the palladium/copper-catalyzed coupling and cyclization of terminal acetylenes and unsaturated imines. This method has been employed to achieve the total synthesis of this compound in seven steps with a 20% overall yield .
Industrial Production Methods
While specific industrial production methods for this compound are not extensively documented, the synthetic routes mentioned above provide a foundation for potential large-scale production. The use of catalytic processes and commercially available starting materials suggests that industrial synthesis could be feasible with further optimization.
化学反応の分析
Types of Reactions
Decumbenine B undergoes various chemical reactions, including:
Oxidation: The compound can be oxidized under specific conditions to form different oxidation products.
Reduction: Reduction reactions can be employed to modify the functional groups within the molecule.
Substitution: Substitution reactions, particularly involving the isoquinoline ring, are common.
Common Reagents and Conditions
Oxidation: Common oxidizing agents include potassium permanganate and chromium trioxide.
Reduction: Reducing agents such as lithium aluminum hydride and sodium borohydride are often used.
Substitution: Reagents like halogens and nucleophiles are employed under various conditions to achieve substitution reactions.
Major Products Formed
The major products formed from these reactions depend on the specific conditions and reagents used. For example, oxidation can lead to the formation of hydroxylated derivatives, while reduction can yield deoxygenated products.
科学的研究の応用
Decumbenine B has a wide range of scientific research applications:
Chemistry: It serves as a model compound for studying isoquinoline alkaloids and their synthetic pathways.
Biology: Research has focused on its biological activity, including its potential as an anti-inflammatory and analgesic agent.
Industry: Its unique chemical structure makes it a valuable compound for developing new pharmaceuticals and chemical intermediates.
作用機序
The mechanism of action of Decumbenine B involves its interaction with specific molecular targets and pathways. It is believed to exert its effects through the modulation of neurotransmitter systems and inhibition of inflammatory pathways. The exact molecular targets and pathways are still under investigation, but initial studies suggest that it may interact with receptors and enzymes involved in pain and inflammation .
類似化合物との比較
Decumbenine B is part of a family of isoquinoline alkaloids, which includes compounds such as:
Decumbenine C: Another isoquinoline alkaloid isolated from Corydalis decumbens, with similar biological activities.
Tetrahydropalmatine: Known for its analgesic and sedative properties.
Bicuculline: A well-known GABA receptor antagonist.
Allocryptopine: Exhibits antispasmodic and analgesic effects.
Bulbocapine: Used for its sedative and muscle relaxant properties.
This compound stands out due to its unique structure and specific therapeutic potential, particularly in the treatment of hypertension and neuralgia .
生物活性
Decumbenine B is an isoquinoline alkaloid that has garnered attention for its diverse biological activities, particularly in the fields of antimicrobial and anticancer research. This article explores the synthesis, biological properties, and potential therapeutic applications of this compound, drawing from various studies and findings.
1. Synthesis of this compound
The total synthesis of this compound has been achieved through several innovative methods, primarily utilizing transition metal-catalyzed reactions. Notably, a Ru(III)-catalyzed C–H activation method has been reported, which allows for the efficient synthesis of this compound from commercially available materials in just five steps with an overall yield of 26.1% .
Table 1: Summary of Synthesis Methods
Method | Catalyst | Steps | Yield (%) |
---|---|---|---|
Ru(III)-catalyzed C–H activation | Ruthenium (Ru) | 5 | 26.1 |
Sequential Heck-Heck reactions | Palladium (Pd) | TBD | TBD |
2. Biological Activity
This compound exhibits a range of biological activities that make it a candidate for further pharmacological studies.
Antimicrobial Activity
Research indicates that this compound has significant antimicrobial properties against various bacterial and fungal strains. Its effectiveness as an antimicrobial agent suggests potential applications in treating infections caused by resistant pathogens.
Anticancer Properties
Recent studies have highlighted the anticancer effects of this compound, particularly against hepatocellular carcinoma (HCC). In vitro studies demonstrated that this compound can inhibit the proliferation of HCC cells, indicating its potential as a therapeutic agent in cancer treatment .
While the exact mechanisms remain under investigation, preliminary data suggest that this compound may exert its effects through modulation of key signaling pathways involved in cell proliferation and apoptosis. For instance, it has been associated with the inhibition of histone deacetylases (HDACs), which play a crucial role in cancer cell survival and proliferation .
3. Case Studies
Several case studies have explored the biological activity of this compound:
- Study on Antimicrobial Effects : A study demonstrated that this compound effectively inhibited the growth of multiple bacterial strains, including both Gram-positive and Gram-negative bacteria. The minimum inhibitory concentration (MIC) values were found to be comparable to those of established antibiotics.
- Hepatocellular Carcinoma Research : In a controlled study involving HCC cell lines, treatment with this compound resulted in a dose-dependent decrease in cell viability. The study also noted morphological changes consistent with apoptosis, further supporting its potential as an anticancer agent .
4. Future Directions
The promising biological activities of this compound warrant further investigation into its pharmacokinetics and safety profile. Future research should focus on:
- Clinical Trials : Conducting clinical trials to evaluate the efficacy and safety of this compound in humans.
- Mechanistic Studies : Elucidating the precise mechanisms through which this compound exerts its biological effects.
- Structural Modifications : Exploring structural analogues to enhance potency and selectivity against specific targets.
特性
IUPAC Name |
[5-([1,3]dioxolo[4,5-f]isoquinolin-8-yl)-1,3-benzodioxol-4-yl]methanol | |
---|---|---|
Source | PubChem | |
URL | https://pubchem.ncbi.nlm.nih.gov | |
Description | Data deposited in or computed by PubChem | |
InChI |
InChI=1S/C18H13NO5/c20-7-13-11(2-4-16-18(13)24-9-22-16)14-5-12-10(6-19-14)1-3-15-17(12)23-8-21-15/h1-6,20H,7-9H2 | |
Source | PubChem | |
URL | https://pubchem.ncbi.nlm.nih.gov | |
Description | Data deposited in or computed by PubChem | |
InChI Key |
GKOMWDNIMJHCDB-UHFFFAOYSA-N | |
Source | PubChem | |
URL | https://pubchem.ncbi.nlm.nih.gov | |
Description | Data deposited in or computed by PubChem | |
Canonical SMILES |
C1OC2=C(O1)C3=CC(=NC=C3C=C2)C4=C(C5=C(C=C4)OCO5)CO | |
Source | PubChem | |
URL | https://pubchem.ncbi.nlm.nih.gov | |
Description | Data deposited in or computed by PubChem | |
Molecular Formula |
C18H13NO5 | |
Source | PubChem | |
URL | https://pubchem.ncbi.nlm.nih.gov | |
Description | Data deposited in or computed by PubChem | |
DSSTOX Substance ID |
DTXSID501166636 | |
Record name | 5-(1,3-Dioxolo[4,5-f]isoquinolin-8-yl)-1,3-benzodioxole-4-methanol | |
Source | EPA DSSTox | |
URL | https://comptox.epa.gov/dashboard/DTXSID501166636 | |
Description | DSSTox provides a high quality public chemistry resource for supporting improved predictive toxicology. | |
Molecular Weight |
323.3 g/mol | |
Source | PubChem | |
URL | https://pubchem.ncbi.nlm.nih.gov | |
Description | Data deposited in or computed by PubChem | |
CAS No. |
164991-68-8 | |
Record name | 5-(1,3-Dioxolo[4,5-f]isoquinolin-8-yl)-1,3-benzodioxole-4-methanol | |
Source | CAS Common Chemistry | |
URL | https://commonchemistry.cas.org/detail?cas_rn=164991-68-8 | |
Description | CAS Common Chemistry is an open community resource for accessing chemical information. Nearly 500,000 chemical substances from CAS REGISTRY cover areas of community interest, including common and frequently regulated chemicals, and those relevant to high school and undergraduate chemistry classes. This chemical information, curated by our expert scientists, is provided in alignment with our mission as a division of the American Chemical Society. | |
Explanation | The data from CAS Common Chemistry is provided under a CC-BY-NC 4.0 license, unless otherwise stated. | |
Record name | 5-(1,3-Dioxolo[4,5-f]isoquinolin-8-yl)-1,3-benzodioxole-4-methanol | |
Source | EPA DSSTox | |
URL | https://comptox.epa.gov/dashboard/DTXSID501166636 | |
Description | DSSTox provides a high quality public chemistry resource for supporting improved predictive toxicology. | |
Retrosynthesis Analysis
AI-Powered Synthesis Planning: Our tool employs the Template_relevance Pistachio, Template_relevance Bkms_metabolic, Template_relevance Pistachio_ringbreaker, Template_relevance Reaxys, Template_relevance Reaxys_biocatalysis model, leveraging a vast database of chemical reactions to predict feasible synthetic routes.
One-Step Synthesis Focus: Specifically designed for one-step synthesis, it provides concise and direct routes for your target compounds, streamlining the synthesis process.
Accurate Predictions: Utilizing the extensive PISTACHIO, BKMS_METABOLIC, PISTACHIO_RINGBREAKER, REAXYS, REAXYS_BIOCATALYSIS database, our tool offers high-accuracy predictions, reflecting the latest in chemical research and data.
Strategy Settings
Precursor scoring | Relevance Heuristic |
---|---|
Min. plausibility | 0.01 |
Model | Template_relevance |
Template Set | Pistachio/Bkms_metabolic/Pistachio_ringbreaker/Reaxys/Reaxys_biocatalysis |
Top-N result to add to graph | 6 |
Feasible Synthetic Routes
Q1: What is Decumbenine B and where is it found?
A1: this compound is a 3-arylisoquinoline alkaloid originally isolated from the roots of the plant Corydalis decumbens. []
Q2: Can you describe the structural characteristics of this compound?
A2: While the provided abstracts do not explicitly state the molecular weight or spectroscopic data, they do offer information about its structure:
- Molecular Formula: C18H13NO4 []
- Structural Features: this compound is characterized by a 3-arylisoquinoline core, meaning an isoquinoline ring system with an aryl group attached at the 3-position. Additionally, it contains a methylenedioxy group, as indicated by the synthetic approaches using 5,6-(methylenedioxy)isoquinoline as a starting material. [, ]
Q3: What are the common synthetic approaches to produce this compound?
A3: Several synthetic routes have been explored for this compound:
- Indiolizine Ring Formation and Cleavage: This method involves creating a dibenz[a,f]indolizin-5(7H)-one ring system, followed by selective cleavage at the amide bond to yield this compound. []
- Palladium/Copper-Catalyzed Coupling and Cyclization: This approach utilizes terminal acetylenes and unsaturated imines. Palladium catalysis facilitates the coupling, while copper catalyzes the subsequent cyclization, leading to the formation of the isoquinoline structure. [, ]
- Ru(III)-Catalyzed C–H Activation: This relatively efficient method focuses on direct ortho-hydroxymethylation of a key intermediate using a Ruthenium catalyst. This approach streamlines the synthesis, offering a shorter route with a good overall yield. []
- Gold(I)-Catalyzed Cascade Reaction: This method employs a gold catalyst to promote a cascade reaction involving cyclization, enyne cycloisomerization, and 1,2-migration, resulting in the formation of a this compound analogue. []
Q4: Has the synthesis of this compound analogues been reported?
A4: Yes, the development of a gold(I)-catalyzed cascade reaction has enabled the synthesis of a this compound analogue. This approach highlights the potential for exploring structural modifications to the this compound scaffold. []
試験管内研究製品の免責事項と情報
BenchChemで提示されるすべての記事および製品情報は、情報提供を目的としています。BenchChemで購入可能な製品は、生体外研究のために特別に設計されています。生体外研究は、ラテン語の "in glass" に由来し、生物体の外で行われる実験を指します。これらの製品は医薬品または薬として分類されておらず、FDAから任何の医療状態、病気、または疾患の予防、治療、または治癒のために承認されていません。これらの製品を人間または動物に体内に導入する形態は、法律により厳格に禁止されています。これらのガイドラインに従うことは、研究と実験において法的および倫理的な基準の遵守を確実にするために重要です。