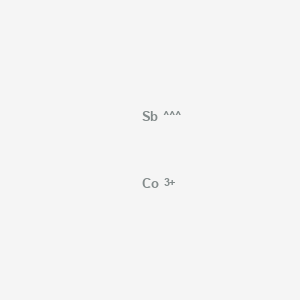
Cobalt antimonide
- 専門家チームからの見積もりを受け取るには、QUICK INQUIRYをクリックしてください。
- 品質商品を競争力のある価格で提供し、研究に集中できます。
説明
Cobalt antimonide (CoSb3) is a skutterudite that has been widely studied for its thermoelectric properties . It can be doped with different elements to further increase its thermopower . By varying the dopant element, it can be tuned to exhibit either p- or n-type semiconductor properties .
Synthesis Analysis
Cobalt antimonide can be synthesized using a solvothermal technique with water as a solvent . The recipe is optimized for the preparation of refined CoSb3 compounds, and a high processing temperature of 500°C and a long duration of 72 hours indicate the presence of the CoSb3 phase .The EDX estimates the elemental composition of Co and Sb in a 1:3 stoichiometric ratio for the as-synthesized CoSb3 .
Physical And Chemical Properties Analysis
Cobalt antimonide is known for its good electrical conductivity and thermopower combined with relatively low thermal conductivity . Both components of the compound, cobalt and antimony, exhibit high affinity for oxygen . Several binary and ternary oxide phases can be formed at elevated temperatures, and some of the oxidation products are volatile, which implies significant material losses .科学的研究の応用
Thermoelectric Materials
Cobalt antimonide is extensively studied for its potential as a thermoelectric material . It exhibits high carrier mobility, high electrical conductivity, and a notably good Seebeck coefficient, making it suitable for converting temperature differences into electric voltage .
Energy Storage Devices
The compound is also advantageous in electrochemical energy storage devices . Compounding cobalt nano-compounds with other materials to construct different structures has shown significant improvements in the electrochemical performance of single cobalt nano-materials .
Nanotechnology
In nanotechnology, cobalt antimonide is used to create nanostructures that enhance performance in various applications, including energy challenges and thermoelectrics .
Automobile Industries
The thermoelectric properties of cobalt antimonide make it applicable in the automobile industry , where it can be used for energy recovery and efficiency improvement .
Portable Electronic Devices
Due to its thermoelectric characteristics, cobalt antimonide can be utilized in portable electronic devices for better energy management and heat dissipation .
Aerospace Industry
Similar to its use in automobiles, cobalt antimonide’s thermoelectric capabilities are also beneficial in the aerospace industry , contributing to energy conservation and thermal management .
作用機序
Target of Action
Cobalt antimonide, also known as antimony; cobalt(3+), is primarily used in the field of materials science, particularly in thermoelectric applications
Mode of Action
The mode of action of cobalt antimonide is primarily physical rather than biological. It’s known for its attractive thermoelectric properties, i.e., good electrical conductivity and thermopower combined with relatively low thermal conductivity . This makes it a potential candidate for use in thermoelectric devices such as power generators or coolers .
Biochemical Pathways
As a material used in thermoelectric applications, cobalt antimonide doesn’t interact with biochemical pathways in the traditional sense. Cobalt, one of the components of cobalt antimonide, is part of the cobalamin molecule (vitamin b12), which plays a crucial role in various biochemical pathways .
Result of Action
The primary result of cobalt antimonide’s action is its impact on thermoelectric performance. It’s known for its good electrical conductivity and thermopower, combined with relatively low thermal conductivity . This makes it a potential candidate for use in thermoelectric devices such as power generators or coolers .
Action Environment
The action of cobalt antimonide is influenced by environmental factors such as temperature and pressure. For instance, the thermoelectric properties of cobalt antimonide can be affected by the sintering temperature .
Safety and Hazards
Cobalt antimonide is classified as Acute toxicity, Oral (Category 3), H301 Acute toxicity, Inhalation (Category 4), H332 Acute toxicity, Dermal (Category 3), H311 Skin irritation (Category 2), H315 Eye irritation (Category 2A), H319 Carcinogenicity (Category 2), H351 Specific target organ toxicity - single exposure (Category 3), Respiratory system, H335 Short-term (acute) aquatic hazard (Category 2), H401 Long-term (chronic) aquatic hazard (Category 2), H411 .
将来の方向性
The currently prepared nanosized skutterudite CoSb3 material synthesized by the solvothermal method could be utilized as active material for the development of highly efficient thermoelectric devices . Numerous efforts have been undertaken to prevent degradation of cobalt triantimonide in service conditions . The best protection developed so far is provided by an aerogel coating, effective in the conditions of dynamic vacuum at temperatures not exceeding 450°C .
特性
IUPAC Name |
antimony;cobalt(3+) |
Source
|
---|---|---|
Source | PubChem | |
URL | https://pubchem.ncbi.nlm.nih.gov | |
Description | Data deposited in or computed by PubChem | |
InChI |
InChI=1S/Co.Sb/q+3; |
Source
|
Source | PubChem | |
URL | https://pubchem.ncbi.nlm.nih.gov | |
Description | Data deposited in or computed by PubChem | |
InChI Key |
NSRGWYQTFLSLOJ-UHFFFAOYSA-N |
Source
|
Source | PubChem | |
URL | https://pubchem.ncbi.nlm.nih.gov | |
Description | Data deposited in or computed by PubChem | |
Canonical SMILES |
[Co+3].[Sb] |
Source
|
Source | PubChem | |
URL | https://pubchem.ncbi.nlm.nih.gov | |
Description | Data deposited in or computed by PubChem | |
Molecular Formula |
CoSb+3 |
Source
|
Source | PubChem | |
URL | https://pubchem.ncbi.nlm.nih.gov | |
Description | Data deposited in or computed by PubChem | |
Molecular Weight |
180.693 g/mol |
Source
|
Source | PubChem | |
URL | https://pubchem.ncbi.nlm.nih.gov | |
Description | Data deposited in or computed by PubChem | |
CAS RN |
12052-42-5 |
Source
|
Record name | Antimony, compd. with cobalt (1:1) | |
Source | ChemIDplus | |
URL | https://pubchem.ncbi.nlm.nih.gov/substance/?source=chemidplus&sourceid=0012052425 | |
Description | ChemIDplus is a free, web search system that provides access to the structure and nomenclature authority files used for the identification of chemical substances cited in National Library of Medicine (NLM) databases, including the TOXNET system. | |
Record name | Antimony, compd. with cobalt (1:1) | |
Source | EPA Chemicals under the TSCA | |
URL | https://www.epa.gov/chemicals-under-tsca | |
Description | EPA Chemicals under the Toxic Substances Control Act (TSCA) collection contains information on chemicals and their regulations under TSCA, including non-confidential content from the TSCA Chemical Substance Inventory and Chemical Data Reporting. | |
Record name | Antimony, compound with cobalt (1:1) | |
Source | European Chemicals Agency (ECHA) | |
URL | https://echa.europa.eu/substance-information/-/substanceinfo/100.031.800 | |
Description | The European Chemicals Agency (ECHA) is an agency of the European Union which is the driving force among regulatory authorities in implementing the EU's groundbreaking chemicals legislation for the benefit of human health and the environment as well as for innovation and competitiveness. | |
Explanation | Use of the information, documents and data from the ECHA website is subject to the terms and conditions of this Legal Notice, and subject to other binding limitations provided for under applicable law, the information, documents and data made available on the ECHA website may be reproduced, distributed and/or used, totally or in part, for non-commercial purposes provided that ECHA is acknowledged as the source: "Source: European Chemicals Agency, http://echa.europa.eu/". Such acknowledgement must be included in each copy of the material. ECHA permits and encourages organisations and individuals to create links to the ECHA website under the following cumulative conditions: Links can only be made to webpages that provide a link to the Legal Notice page. | |
試験管内研究製品の免責事項と情報
BenchChemで提示されるすべての記事および製品情報は、情報提供を目的としています。BenchChemで購入可能な製品は、生体外研究のために特別に設計されています。生体外研究は、ラテン語の "in glass" に由来し、生物体の外で行われる実験を指します。これらの製品は医薬品または薬として分類されておらず、FDAから任何の医療状態、病気、または疾患の予防、治療、または治癒のために承認されていません。これらの製品を人間または動物に体内に導入する形態は、法律により厳格に禁止されています。これらのガイドラインに従うことは、研究と実験において法的および倫理的な基準の遵守を確実にするために重要です。