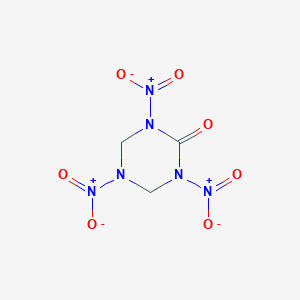
1,3,5-Trinitro-1,3,5-triazinan-2-one
カタログ番号:
B169740
CAS番号:
115029-35-1
分子量:
236.1 g/mol
InChIキー:
UBBZKFUIBVLJCP-UHFFFAOYSA-N
注意:
研究専用です。人間または獣医用ではありません。
在庫あり
- 専門家チームからの見積もりを受け取るには、QUICK INQUIRYをクリックしてください。
- 品質商品を競争力のある価格で提供し、研究に集中できます。
説明
1,3,5-Trinitro-1,3,5-triazinan-2-one, also known as RDX, is a relatively stable molecule that releases a large amount of energy upon decomposition . It has a molecular formula of C3H4N6O7, an average mass of 236.100 Da, and a monoisotopic mass of 236.014145 Da . It’s widely used in both industrial and military explosives .
Molecular Structure Analysis
The molecular structure of this compound is complex, with a molecular formula of CHNO, an average mass of 236.100 Da, and a monoisotopic mass of 236.014145 Da .Chemical Reactions Analysis
Reactive molecular dynamics simulations have been performed to study the decomposition processes of this compound under high temperatures and detonation pressure . The initial decomposition paths are similar, which is due to the N–NO2 bond breakage to release NO2 .Physical And Chemical Properties Analysis
The physical and chemical properties of this compound include a molecular formula of CHNO, an average mass of 236.100 Da, and a monoisotopic mass of 236.014145 Da .作用機序
将来の方向性
特性
IUPAC Name |
1,3,5-trinitro-1,3,5-triazinan-2-one |
Source
|
---|---|---|
Source | PubChem | |
URL | https://pubchem.ncbi.nlm.nih.gov | |
Description | Data deposited in or computed by PubChem | |
InChI |
InChI=1S/C3H4N6O7/c10-3-5(8(13)14)1-4(7(11)12)2-6(3)9(15)16/h1-2H2 |
Source
|
Source | PubChem | |
URL | https://pubchem.ncbi.nlm.nih.gov | |
Description | Data deposited in or computed by PubChem | |
InChI Key |
UBBZKFUIBVLJCP-UHFFFAOYSA-N |
Source
|
Source | PubChem | |
URL | https://pubchem.ncbi.nlm.nih.gov | |
Description | Data deposited in or computed by PubChem | |
Canonical SMILES |
C1N(CN(C(=O)N1[N+](=O)[O-])[N+](=O)[O-])[N+](=O)[O-] |
Source
|
Source | PubChem | |
URL | https://pubchem.ncbi.nlm.nih.gov | |
Description | Data deposited in or computed by PubChem | |
Molecular Formula |
C3H4N6O7 |
Source
|
Source | PubChem | |
URL | https://pubchem.ncbi.nlm.nih.gov | |
Description | Data deposited in or computed by PubChem | |
DSSTOX Substance ID |
DTXSID00448887 |
Source
|
Record name | 1,3,5-Trinitro-1,3,5-triazinan-2-one | |
Source | EPA DSSTox | |
URL | https://comptox.epa.gov/dashboard/DTXSID00448887 | |
Description | DSSTox provides a high quality public chemistry resource for supporting improved predictive toxicology. | |
Molecular Weight |
236.10 g/mol |
Source
|
Source | PubChem | |
URL | https://pubchem.ncbi.nlm.nih.gov | |
Description | Data deposited in or computed by PubChem | |
CAS No. |
115029-35-1 |
Source
|
Record name | 1,3,5-Trinitro-1,3,5-triazinan-2-one | |
Source | EPA DSSTox | |
URL | https://comptox.epa.gov/dashboard/DTXSID00448887 | |
Description | DSSTox provides a high quality public chemistry resource for supporting improved predictive toxicology. | |
試験管内研究製品の免責事項と情報
BenchChemで提示されるすべての記事および製品情報は、情報提供を目的としています。BenchChemで購入可能な製品は、生体外研究のために特別に設計されています。生体外研究は、ラテン語の "in glass" に由来し、生物体の外で行われる実験を指します。これらの製品は医薬品または薬として分類されておらず、FDAから任何の医療状態、病気、または疾患の予防、治療、または治癒のために承認されていません。これらの製品を人間または動物に体内に導入する形態は、法律により厳格に禁止されています。これらのガイドラインに従うことは、研究と実験において法的および倫理的な基準の遵守を確実にするために重要です。
迅速なお問い合わせ
カテゴリー
最も閲覧された
Methyl 6-amino-7-methoxy-2H-1,3-benzodioxole-5-carboxyl...
Cat. No.: B169657
CAS No.: 109893-05-2
4-(2-Chloroethyl)-1-(triphenylmethyl)-1H-imidazole
Cat. No.: B169658
CAS No.: 132287-55-9
4-(1H-Imidazol-1-YL)-3-nitrobenzoic acid
Cat. No.: B169659
CAS No.: 167626-67-7
6-Methyl-1H-pyrazolo[4,3-c]pyridine
Cat. No.: B169660
CAS No.: 1357946-60-1