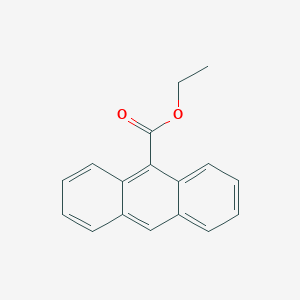
9-アントラセンカルボン酸エチルエステル
概要
説明
Ethyl anthracene-9-carboxylate is an organic compound with the molecular formula C17H14O2. It is an ester derivative of anthracene-9-carboxylic acid, where the carboxyl group is esterified with an ethyl group. This compound is known for its aromatic structure, which includes a three-ring anthracene system, making it a subject of interest in various chemical research and industrial applications.
科学的研究の応用
Ethyl anthracene-9-carboxylate has a wide range of applications in scientific research:
Chemistry: It is used as a precursor in the synthesis of various anthracene derivatives, which are important in the study of photophysical and photochemical properties.
Biology: The compound is investigated for its potential biological activities, including antimicrobial and anticancer properties.
Medicine: Research is ongoing to explore its potential as a therapeutic agent, particularly in the development of drugs targeting specific molecular pathways.
Industry: It is used in the production of dyes, pigments, and organic semiconductors due to its stable aromatic structure and electronic properties.
作用機序
Target of Action
Ethyl anthracene-9-carboxylate is a derivative of 9-anthracene carboxylic acid (9-AC), a rigid organic molecule commonly utilized in the construction of coordination complexes .
Mode of Action
The compound exhibits radical-induced photochromism and photomagnetism upon irradiation with Xe lamp light . This light-actuated behavior is crucial for the design of new photochromic complexes and optical switching devices . The compound’s interaction with light leads to the generation of stable radicals, which are important in the field of optical switching, displays, and other devices .
Biochemical Pathways
The biochemical pathways affected by ethyl anthracene-9-carboxylate are primarily related to the photogeneration of stable radicals . The compound is prone to intermolecular [4+4] cycloaddition reactions after UV light irradiation, inducing mechanical bending, magnetic, and photoluminescent variations . The dimer analogue could also be reversibly returned to the monomer state via either a heat treatment or another light irradiation .
Pharmacokinetics
The compound’s large π-conjugated structure may influence its bioavailability and stability .
Result of Action
The molecular and cellular effects of ethyl anthracene-9-carboxylate’s action are largely dependent on its interaction with light. Upon light irradiation, the compound undergoes a color change, which has been naked-eye detected in some anthracene-based complexes . This color change is due to the generation of stable radicals, which can be used in the construction of photochromic materials .
Action Environment
Environmental factors, such as light and temperature, significantly influence the action, efficacy, and stability of ethyl anthracene-9-carboxylate. For instance, the compound shows radical-induced photochromism and photomagnetism after Xe lamp light irradiation . Furthermore, the dimer analogue of the compound can be reversibly returned to the monomer state via either a heat treatment or another light irradiation .
生化学分析
Biochemical Properties
Ethyl anthracene-9-carboxylate has been found to interact with various enzymes and proteins. For instance, it has been shown to interact with enzymes such as manganese peroxidase (MnP), lignin peroxidase (LiP), laccase, 1,2-dioxygenase, and 2,3-dioxgenase . These interactions are crucial for its role in biochemical reactions, particularly in the field of optical switching, displays, and other devices .
Cellular Effects
It has been suggested that it may influence cell function through its impact on cell signaling pathways, gene expression, and cellular metabolism
Molecular Mechanism
The molecular mechanism of action of ethyl anthracene-9-carboxylate involves its ability to generate stable radicals. This process is important in the field of optical switching, displays, and other devices . It is also suggested that it exerts its effects at the molecular level through binding interactions with biomolecules, enzyme inhibition or activation, and changes in gene expression .
Temporal Effects in Laboratory Settings
The effects of ethyl anthracene-9-carboxylate over time in laboratory settings have been observed. It has been found that the photogeneration of stable radicals is a light-induced electron transfer process, and the stable radicals are produced after Xe lamp illumination
Metabolic Pathways
It is known that it interacts with enzymes such as manganese peroxidase (MnP), lignin peroxidase (LiP), laccase, 1,2-dioxygenase, and 2,3-dioxgenase . These interactions could potentially affect metabolic flux or metabolite levels.
Transport and Distribution
It is possible that it interacts with transporters or binding proteins, which could affect its localization or accumulation .
Subcellular Localization
It is possible that it has targeting signals or post-translational modifications that direct it to specific compartments or organelles .
準備方法
Synthetic Routes and Reaction Conditions: The synthesis of ethyl anthracene-9-carboxylate typically involves the esterification of anthracene-9-carboxylic acid with ethanol. The reaction is usually catalyzed by an acid, such as sulfuric acid or hydrochloric acid, under reflux conditions. The general reaction can be represented as follows:
Anthracene-9-carboxylic acid+EthanolAcid catalystEthyl anthracene-9-carboxylate+Water
Industrial Production Methods: In an industrial setting, the production of ethyl anthracene-9-carboxylate may involve continuous flow processes to enhance efficiency and yield. The use of advanced catalysts and optimized reaction conditions can further improve the scalability of the synthesis.
Types of Reactions:
Oxidation: Ethyl anthracene-9-carboxylate can undergo oxidation reactions, typically using strong oxidizing agents like potassium permanganate or chromium trioxide, leading to the formation of anthraquinone derivatives.
Reduction: Reduction of ethyl anthracene-9-carboxylate can be achieved using reducing agents such as lithium aluminum hydride, resulting in the formation of anthracene-9-methanol derivatives.
Substitution: The aromatic rings of ethyl anthracene-9-carboxylate can participate in electrophilic substitution reactions, such as nitration, sulfonation, and halogenation, using reagents like nitric acid, sulfuric acid, and halogens, respectively.
Common Reagents and Conditions:
Oxidation: Potassium permanganate in an alkaline medium.
Reduction: Lithium aluminum hydride in anhydrous ether.
Substitution: Concentrated nitric acid for nitration, concentrated sulfuric acid for sulfonation, and halogens (chlorine, bromine) for halogenation.
Major Products:
Oxidation: Anthraquinone derivatives.
Reduction: Anthracene-9-methanol derivatives.
Substitution: Nitro, sulfo, and halo derivatives of ethyl anthracene-9-carboxylate.
類似化合物との比較
Anthracene-9-carboxylic acid: The parent compound, which lacks the ethyl ester group.
Ethyl anthracene-9-methanol: A reduced form of ethyl anthracene-9-carboxylate.
Anthraquinone derivatives: Oxidized forms of anthracene compounds.
Uniqueness: Ethyl anthracene-9-carboxylate is unique due to its ester functional group, which imparts different chemical reactivity and physical properties compared to its parent acid and other derivatives. The presence of the ethyl group enhances its solubility in organic solvents and can influence its interaction with biological targets.
生物活性
Ethyl anthracene-9-carboxylate (EAC) is an organic compound with the molecular formula C17H14O2, recognized for its potential biological activities, particularly in antimicrobial and anticancer research. This article explores the various aspects of its biological activity, including mechanisms of action, biochemical interactions, and relevant case studies.
Overview of Ethyl Anthracene-9-Carboxylate
EAC is an ester derivative of anthracene-9-carboxylic acid, characterized by its aromatic structure that includes a three-ring anthracene system. Its unique properties have led to investigations into its applications in both medicinal chemistry and materials science.
1. Photochemical Properties:
EAC exhibits radical-induced photochromism and photomagnetism when irradiated with light. This property is significant for applications in optical devices and materials science. The compound undergoes a color change upon light exposure, which can be detected visually, indicating its potential use in photonic applications.
2. Interaction with Enzymes:
EAC has been shown to interact with various enzymes, including:
- Manganese Peroxidase (MnP)
- Lignin Peroxidase (LiP)
- Laccase
- 1,2-Dioxygenase
- 2,3-Dioxygenase
These interactions suggest that EAC may influence metabolic pathways and cellular functions through enzyme modulation .
EAC's biochemical properties are critical to understanding its biological activity:
Property | Description |
---|---|
Molecular Weight | 258.29 g/mol |
Solubility | Soluble in organic solvents; limited solubility in water |
Stability | Stable under standard laboratory conditions; sensitive to light |
The compound's large π-conjugated structure may affect its bioavailability and stability, which are important factors in pharmacokinetics.
Biological Activity
1. Antimicrobial Activity:
Research indicates that EAC possesses antimicrobial properties. It has been tested against various bacterial strains, demonstrating inhibitory effects that warrant further exploration for potential therapeutic applications.
2. Anticancer Properties:
EAC has shown promise in anticancer research, particularly in screenings against the National Cancer Institute's 60 cell line panel. Preliminary studies suggest that it may exhibit cytotoxic effects comparable to established chemotherapeutic agents .
Case Studies
Study 1: Anticancer Screening
In a study evaluating the anticancer activity of EAC, researchers found that it induced apoptosis in cancer cell lines through the generation of reactive oxygen species (ROS). This mechanism highlights the compound's potential as a lead candidate for drug development targeting specific molecular pathways involved in tumor growth .
Study 2: Enzyme Interaction Analysis
A detailed analysis of EAC's interaction with MnP revealed that it could enhance the enzyme's activity, suggesting a role in lignin degradation processes. This finding has implications for bioremediation and biofuel production .
特性
IUPAC Name |
ethyl anthracene-9-carboxylate | |
---|---|---|
Source | PubChem | |
URL | https://pubchem.ncbi.nlm.nih.gov | |
Description | Data deposited in or computed by PubChem | |
InChI |
InChI=1S/C17H14O2/c1-2-19-17(18)16-14-9-5-3-7-12(14)11-13-8-4-6-10-15(13)16/h3-11H,2H2,1H3 | |
Source | PubChem | |
URL | https://pubchem.ncbi.nlm.nih.gov | |
Description | Data deposited in or computed by PubChem | |
InChI Key |
HAIGJDFIKOFIAB-UHFFFAOYSA-N | |
Source | PubChem | |
URL | https://pubchem.ncbi.nlm.nih.gov | |
Description | Data deposited in or computed by PubChem | |
Canonical SMILES |
CCOC(=O)C1=C2C=CC=CC2=CC3=CC=CC=C31 | |
Source | PubChem | |
URL | https://pubchem.ncbi.nlm.nih.gov | |
Description | Data deposited in or computed by PubChem | |
Molecular Formula |
C17H14O2 | |
Source | PubChem | |
URL | https://pubchem.ncbi.nlm.nih.gov | |
Description | Data deposited in or computed by PubChem | |
DSSTOX Substance ID |
DTXSID80505568 | |
Record name | Ethyl anthracene-9-carboxylate | |
Source | EPA DSSTox | |
URL | https://comptox.epa.gov/dashboard/DTXSID80505568 | |
Description | DSSTox provides a high quality public chemistry resource for supporting improved predictive toxicology. | |
Molecular Weight |
250.29 g/mol | |
Source | PubChem | |
URL | https://pubchem.ncbi.nlm.nih.gov | |
Description | Data deposited in or computed by PubChem | |
CAS No. |
1754-54-7 | |
Record name | Ethyl anthracene-9-carboxylate | |
Source | EPA DSSTox | |
URL | https://comptox.epa.gov/dashboard/DTXSID80505568 | |
Description | DSSTox provides a high quality public chemistry resource for supporting improved predictive toxicology. | |
Retrosynthesis Analysis
AI-Powered Synthesis Planning: Our tool employs the Template_relevance Pistachio, Template_relevance Bkms_metabolic, Template_relevance Pistachio_ringbreaker, Template_relevance Reaxys, Template_relevance Reaxys_biocatalysis model, leveraging a vast database of chemical reactions to predict feasible synthetic routes.
One-Step Synthesis Focus: Specifically designed for one-step synthesis, it provides concise and direct routes for your target compounds, streamlining the synthesis process.
Accurate Predictions: Utilizing the extensive PISTACHIO, BKMS_METABOLIC, PISTACHIO_RINGBREAKER, REAXYS, REAXYS_BIOCATALYSIS database, our tool offers high-accuracy predictions, reflecting the latest in chemical research and data.
Strategy Settings
Precursor scoring | Relevance Heuristic |
---|---|
Min. plausibility | 0.01 |
Model | Template_relevance |
Template Set | Pistachio/Bkms_metabolic/Pistachio_ringbreaker/Reaxys/Reaxys_biocatalysis |
Top-N result to add to graph | 6 |
Feasible Synthetic Routes
Q1: What is the molecular formula and structure of ethyl anthracene-9-carboxylate?
A1: Ethyl anthracene-9-carboxylate has the molecular formula C17H14O2. [] Structurally, it consists of an anthracene moiety with an ethyl carboxylate group substituted at the 9 position. The carboxylate group and the anthracene ring system are not coplanar, with a dihedral angle of 76.00° between them. []
Q2: How does ethyl anthracene-9-carboxylate pack in its crystal structure?
A2: The crystal structure of ethyl anthracene-9-carboxylate is stabilized by a combination of C–H⋯O hydrogen bonds and edge-to-face arene interactions. [] These interactions contribute to the arrangement of molecules within the crystal lattice.
Q3: Has ethyl anthracene-9-carboxylate been used in the synthesis of other materials?
A3: Yes, ethyl anthracene-9-carboxylate has been used as a photoactive monomer in the synthesis of amphiphilic diblock copolymers. [] When copolymerized with poly(ethylene glycol) and linear brush polydimethylsiloxane, it allows for the formation of nanoparticles that can be crosslinked by UV irradiation due to the presence of the anthracene moiety. []
試験管内研究製品の免責事項と情報
BenchChemで提示されるすべての記事および製品情報は、情報提供を目的としています。BenchChemで購入可能な製品は、生体外研究のために特別に設計されています。生体外研究は、ラテン語の "in glass" に由来し、生物体の外で行われる実験を指します。これらの製品は医薬品または薬として分類されておらず、FDAから任何の医療状態、病気、または疾患の予防、治療、または治癒のために承認されていません。これらの製品を人間または動物に体内に導入する形態は、法律により厳格に禁止されています。これらのガイドラインに従うことは、研究と実験において法的および倫理的な基準の遵守を確実にするために重要です。