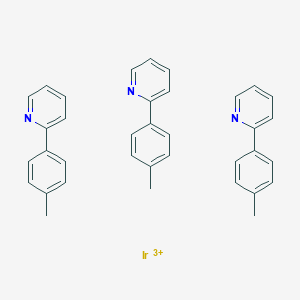
Ir(mppy)3
概要
説明
Tris[2-(p-tolyl)pyridine]iridium(III), commonly referred to as Ir(Mppy)3, is a phosphorescent dopant widely used in organic light-emitting diodes (OLEDs) and thermally activated delayed fluorescence (TADF) OLED devices. This compound emits green light and is known for its high efficiency and stability. The presence of three methyl groups attached to the pyridine ligands enhances its solubility compared to other similar compounds .
科学的研究の応用
Tris[2-(p-tolyl)pyridine]iridium(III) has a wide range of applications in scientific research:
Biology: Investigated for its potential use in bioimaging and as a photosensitizer in photodynamic therapy.
Medicine: Explored for its potential in cancer treatment due to its photophysical properties.
作用機序
Target of Action
The primary target of Ir(mppy)3 is the Organic Light Emitting Diodes (OLEDs) and Thermally Activated Delayed Fluorescence OLEDs (TADF-OLEDs) . It acts as a phosphorescent dopant , emitting green light in these devices .
Mode of Action
This compound interacts with its targets through excitation energy transfer (EET) and charge transfer (CT) mechanisms . These processes are competitive, with the EET mechanism being dominant . The excitons are primarily formed in the host first and then transferred to the guest in phosphorescent OLEDs based on exciplex-forming cohosts .
Biochemical Pathways
The action of this compound affects the energy transfer pathways from the exciplex state of cohost to the emissive state of the guest . Understanding these pathways can provide insights for improving exciplex-forming materials adopted in OLEDs .
Pharmacokinetics
While the term “pharmacokinetics” is typically used in the context of drug metabolism, in the case of this compound, we can discuss its solubility and stability . This compound is more soluble than the widely known green emitter Ir(ppy)3 due to the presence of three methyl groups attached to the ppy ligands . This increased solubility can impact the bioavailability of the compound in the OLED environment.
Result of Action
The result of this compound’s action is the emission of green light in highly efficient OLED and TADF-OLED devices . When co-doped with Ir(ppz)3, this compound devices show a slower efficiency roll-off and higher electroluminescent efficiencies due to improved recombination probability and suppressed exciton quenching .
Action Environment
The action, efficacy, and stability of this compound are influenced by environmental factors. For instance, the relative importance of CT over EET depends strongly on the location of the guest molecule relative to the cohost pair . Both the coupling for EET and the interaction energy for CT are strongly influenced by the geometric constraints .
準備方法
Synthetic Routes and Reaction Conditions
The synthesis of Tris[2-(p-tolyl)pyridine]iridium(III) typically involves the coordination of iridium chloride (IrCl3) with 2-(p-tolyl)pyridine ligands. The reaction is carried out under reflux conditions in the presence of a suitable solvent such as dichloromethane or toluene. The product is then purified through sublimation to achieve high purity levels .
Industrial Production Methods
In industrial settings, the production of Tris[2-(p-tolyl)pyridine]iridium(III) follows similar synthetic routes but on a larger scale. The process involves the use of automated reactors and purification systems to ensure consistency and high yield. The compound is often produced in sublimed form to meet the stringent purity requirements for electronic applications .
化学反応の分析
Types of Reactions
Tris[2-(p-tolyl)pyridine]iridium(III) primarily undergoes photophysical reactions due to its role as a phosphorescent dopant. It can participate in oxidation and reduction reactions, as well as ligand substitution reactions.
Common Reagents and Conditions
Oxidation: Common oxidizing agents include oxygen and hydrogen peroxide.
Reduction: Reducing agents such as sodium borohydride or hydrazine can be used.
Substitution: Ligand substitution reactions often involve the use of other pyridine derivatives or phosphine ligands under reflux conditions
Major Products
The major products formed from these reactions depend on the specific reagents and conditions used. For example, oxidation may lead to the formation of iridium oxides, while substitution reactions can yield various iridium complexes with different ligands .
類似化合物との比較
Similar Compounds
- Tris[2-phenylpyridinato-C2,N]iridium(III) (Ir(ppy)3)
- Tris[2-(4,6-difluorophenyl)pyridinato-C2,N]iridium(III) (Ir(dFppy)3)
- Tris[2-(4-tolyl)pyridinato-N,C2’]iridium(III) (Ir(p-Me-ppy)3)
Uniqueness
Tris[2-(p-tolyl)pyridine]iridium(III) stands out due to its enhanced solubility and higher electroluminescent efficiency compared to other green emitters like Ir(ppy)3. The presence of methyl groups on the pyridine ligands improves its performance in OLED devices by reducing efficiency roll-off and suppressing exciton quenching .
特性
IUPAC Name |
iridium(3+);2-(4-methylphenyl)pyridine | |
---|---|---|
Source | PubChem | |
URL | https://pubchem.ncbi.nlm.nih.gov | |
Description | Data deposited in or computed by PubChem | |
InChI |
InChI=1S/3C12H11N.Ir/c3*1-10-5-7-11(8-6-10)12-4-2-3-9-13-12;/h3*2-9H,1H3;/q;;;+3 | |
Source | PubChem | |
URL | https://pubchem.ncbi.nlm.nih.gov | |
Description | Data deposited in or computed by PubChem | |
InChI Key |
YERGTYJYQCLVDM-UHFFFAOYSA-N | |
Source | PubChem | |
URL | https://pubchem.ncbi.nlm.nih.gov | |
Description | Data deposited in or computed by PubChem | |
Canonical SMILES |
CC1=CC=C(C=C1)C2=CC=CC=N2.CC1=CC=C(C=C1)C2=CC=CC=N2.CC1=CC=C(C=C1)C2=CC=CC=N2.[Ir+3] | |
Source | PubChem | |
URL | https://pubchem.ncbi.nlm.nih.gov | |
Description | Data deposited in or computed by PubChem | |
Molecular Formula |
C36H33IrN3+3 | |
Source | PubChem | |
URL | https://pubchem.ncbi.nlm.nih.gov | |
Description | Data deposited in or computed by PubChem | |
Molecular Weight |
699.9 g/mol | |
Source | PubChem | |
URL | https://pubchem.ncbi.nlm.nih.gov | |
Description | Data deposited in or computed by PubChem | |
Retrosynthesis Analysis
AI-Powered Synthesis Planning: Our tool employs the Template_relevance Pistachio, Template_relevance Bkms_metabolic, Template_relevance Pistachio_ringbreaker, Template_relevance Reaxys, Template_relevance Reaxys_biocatalysis model, leveraging a vast database of chemical reactions to predict feasible synthetic routes.
One-Step Synthesis Focus: Specifically designed for one-step synthesis, it provides concise and direct routes for your target compounds, streamlining the synthesis process.
Accurate Predictions: Utilizing the extensive PISTACHIO, BKMS_METABOLIC, PISTACHIO_RINGBREAKER, REAXYS, REAXYS_BIOCATALYSIS database, our tool offers high-accuracy predictions, reflecting the latest in chemical research and data.
Strategy Settings
Precursor scoring | Relevance Heuristic |
---|---|
Min. plausibility | 0.01 |
Model | Template_relevance |
Template Set | Pistachio/Bkms_metabolic/Pistachio_ringbreaker/Reaxys/Reaxys_biocatalysis |
Top-N result to add to graph | 6 |
Feasible Synthetic Routes
試験管内研究製品の免責事項と情報
BenchChemで提示されるすべての記事および製品情報は、情報提供を目的としています。BenchChemで購入可能な製品は、生体外研究のために特別に設計されています。生体外研究は、ラテン語の "in glass" に由来し、生物体の外で行われる実験を指します。これらの製品は医薬品または薬として分類されておらず、FDAから任何の医療状態、病気、または疾患の予防、治療、または治癒のために承認されていません。これらの製品を人間または動物に体内に導入する形態は、法律により厳格に禁止されています。これらのガイドラインに従うことは、研究と実験において法的および倫理的な基準の遵守を確実にするために重要です。