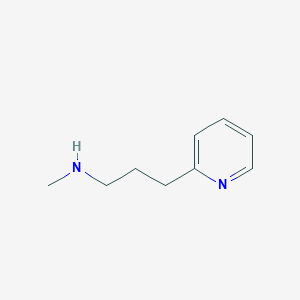
N-Methyl-3-(pyridin-2-yl)propan-1-amine
- 専門家チームからの見積もりを受け取るには、QUICK INQUIRYをクリックしてください。
- 品質商品を競争力のある価格で提供し、研究に集中できます。
説明
“N-Methyl-3-(pyridin-2-yl)propan-1-amine” is a chemical compound . It is related to Chlorpheniramine, a compound used in medicine . The molecular formula of the compound is C15H17ClN2 .
Synthesis Analysis
The synthesis of compounds similar to “N-Methyl-3-(pyridin-2-yl)propan-1-amine” has been reported in the literature . These methods often involve the use of palladium-catalyzed coupling reactions or chemodivergent synthesis from α-bromoketones and 2-aminopyridines .
科学的研究の応用
Synthesis of N-(pyridin-2-yl)amides and 3-bromoimidazo[1,2-a]pyridines
N-Methyl-3-(pyridin-2-yl)propan-1-amine is used in the chemodivergent synthesis of N-(pyridin-2-yl)amides and 3-bromoimidazo[1,2-a]pyridines from α-bromoketones and 2-aminopyridine under different reaction conditions . N-(Pyridin-2-yl)amides were formed in toluene via C–C bond cleavage promoted by I2 and TBHP and the reaction conditions were mild and metal-free .
Synthesis of N-(pyridin-2-yl)imidates
This compound is also used in the synthesis of N-(pyridin-2-yl)imidates from nitrostyrenes and 2-aminopyridines via the corresponding N-(pyridin-2-yl)iminonitriles as intermediates . The reaction process involved the in situ formation of the corresponding α-iminontriles under heterogeneous Lewis acid catalysis in the presence of Al2O3 .
Conversion into N-heterocycles
A preliminary synthetic application of the present N-(pyridin-2-yl)imidates was carried out for their facile conversion into the N-heterocycles 2-(4-chlorophenyl)-4,5-dihydro-1H-imidazole and 2-(4-chlorophenyl)-1,4,5,6-tetrahydropyrimidine in the presence of the corresponding ethylenediamine and 1,3-diaminopropane .
Anticancer Activity
Some compounds derived from N-Methyl-3-(pyridin-2-yl)propan-1-amine have shown excellent antiproliferative activity against certain cell lines, such as the A549 cell line and HCT116 cell line .
Safety and Hazards
作用機序
Mode of Action
It is likely that the compound interacts with its targets through a variety of mechanisms, potentially including binding to receptors or enzymes, altering cellular processes, or disrupting biochemical pathways .
Biochemical Pathways
Given the compound’s structure and potential targets, it may be involved in a variety of pathways related to cellular signaling, metabolism, or other cellular processes .
Pharmacokinetics
These properties would significantly impact the compound’s bioavailability and overall effectiveness .
Result of Action
Given its potential targets and modes of action, it may have a variety of effects at the molecular and cellular levels .
Action Environment
The action of N-Methyl-3-(pyridin-2-yl)propan-1-amine can be influenced by a variety of environmental factors, including pH, temperature, and the presence of other compounds. These factors can affect the compound’s stability, efficacy, and overall action .
特性
IUPAC Name |
N-methyl-3-pyridin-2-ylpropan-1-amine |
Source
|
---|---|---|
Source | PubChem | |
URL | https://pubchem.ncbi.nlm.nih.gov | |
Description | Data deposited in or computed by PubChem | |
InChI |
InChI=1S/C9H14N2/c1-10-7-4-6-9-5-2-3-8-11-9/h2-3,5,8,10H,4,6-7H2,1H3 |
Source
|
Source | PubChem | |
URL | https://pubchem.ncbi.nlm.nih.gov | |
Description | Data deposited in or computed by PubChem | |
InChI Key |
DJIJZMRJLJLWGR-UHFFFAOYSA-N |
Source
|
Source | PubChem | |
URL | https://pubchem.ncbi.nlm.nih.gov | |
Description | Data deposited in or computed by PubChem | |
Canonical SMILES |
CNCCCC1=CC=CC=N1 |
Source
|
Source | PubChem | |
URL | https://pubchem.ncbi.nlm.nih.gov | |
Description | Data deposited in or computed by PubChem | |
Molecular Formula |
C9H14N2 |
Source
|
Source | PubChem | |
URL | https://pubchem.ncbi.nlm.nih.gov | |
Description | Data deposited in or computed by PubChem | |
Molecular Weight |
150.22 g/mol |
Source
|
Source | PubChem | |
URL | https://pubchem.ncbi.nlm.nih.gov | |
Description | Data deposited in or computed by PubChem | |
Product Name |
N-Methyl-3-(pyridin-2-yl)propan-1-amine |
試験管内研究製品の免責事項と情報
BenchChemで提示されるすべての記事および製品情報は、情報提供を目的としています。BenchChemで購入可能な製品は、生体外研究のために特別に設計されています。生体外研究は、ラテン語の "in glass" に由来し、生物体の外で行われる実験を指します。これらの製品は医薬品または薬として分類されておらず、FDAから任何の医療状態、病気、または疾患の予防、治療、または治癒のために承認されていません。これらの製品を人間または動物に体内に導入する形態は、法律により厳格に禁止されています。これらのガイドラインに従うことは、研究と実験において法的および倫理的な基準の遵守を確実にするために重要です。