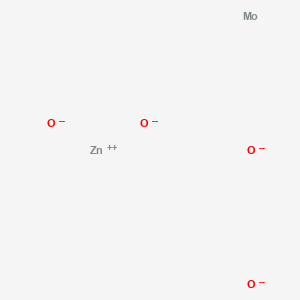
Molybdenum zinc oxide
説明
Molybdenum zinc oxide (ZnMoO₄) is a mixed metal oxide combining zinc oxide (ZnO) and molybdenum oxide (MoO₃). It crystallizes in polymorphic forms, primarily triclinic (α-ZnMoO₄) and monoclinic (β-ZnMoO₄) structures . In the triclinic phase, zinc forms a distorted octahedral complex [ZnO₆], while molybdenum adopts a tetrahedral [MoO₄] configuration. The monoclinic β-phase features both Zn and Mo in octahedral coordination ([ZnO₆]/[MoO₆]), resembling a wolframite-type structure .
ZnMoO₄ exhibits unique optoelectronic properties, including a wide bandgap (~3.43 eV) and high visible-light transmittance (84–87%), making it a promising transparent conducting oxide (TCO) for photovoltaic cells . Its electrical conductivity reaches 168,067 S/m when doped with molybdenum, rivaling traditional materials like indium tin oxide (ITO) . Additionally, Mo-doped ZnO demonstrates enhanced thermoelectric performance (e.g., increased carrier concentration of 3.1×10²⁰ cm⁻³) and mechanical robustness, enabling applications in smart meters and flexible electronics .
Synthesis methods include solid-state reactions (e.g., grinding oxalic acid, ammonium molybdate, and zinc nitrate at 160°C) and solution-based techniques controlling Zn/Mo molar ratios .
準備方法
Hydrothermal Synthesis of Molybdenum-Doped Zinc Oxide
Hydrothermal synthesis is a widely adopted method for producing Mo-ZnO nanostructures due to its ability to control morphology and crystallinity. A representative protocol involves dissolving 0.1 M zinc acetate (Zn(CH₃COO)₂·2H₂O) and 1–5 wt% ammonium molybdate ((NH₄)₆Mo₇O₂₄·4H₂O) in 150 mL of distilled water. Hexamethylenetetramine (C₆H₁₂N₄) and cetyltrimethylammonium bromide are added as structure-directing agents, followed by pH adjustment to 8–9 using sodium hydroxide (NaOH). The mixture is heated in a Teflon-lined autoclave at 180°C for 12 hours, yielding hierarchical nanoflowers (Fig. 1A).
Key Parameters and Outcomes
XRD analysis confirms the hexagonal wurtzite structure of ZnO, with peak shifts indicating successful Mo⁶⁺ incorporation into the lattice. Raman spectroscopy further validates lattice distortion due to Mo doping, while BET analysis reveals a surface area of 6.02 m²/g for nanoflowers, facilitating enhanced catalytic activity.
Solution-Based Synthesis of Zinc Molybdate Compounds
Zinc molybdate (ZnMoO₄) and basic zinc molybdate (ZnMoO₄·xH₂O) are synthesized via aqueous precipitation using industrial-grade zinc oxide (ZnO) and ammonium molybdate. The Zn/Mo molar ratio dictates product composition:
Reaction Conditions and Yield
Zn/Mo Ratio | Temperature | Time | Product | Purity |
---|---|---|---|---|
0.5:1 | 80°C | 6 h | ZnMoO₄ | 99.1% |
2:1 | 60°C | 8 h | ZnMoO₄·2H₂O | 95.8% |
3:1 | 40°C | 12 h | ZnMoO₄·4H₂O | 96.2% |
The process involves dissolving ZnO in ammonium molybdate solution under stirring, followed by centrifugation and drying. Ammonia gas generated during the reaction is absorbed for reuse, enhancing sustainability.
Sol-Gel Synthesis of Mo-ZnO Thin Films
The sol-gel method enables the fabrication of Mo-ZnO thin films for optoelectronic applications. A typical procedure combines zinc acetate dihydrate (Zn(CH₃COO)₂·2H₂O) and ammonium heptamolybdate tetrahydrate in ethanol, with nitric acid as a catalyst. The sol is aged for 24 hours, spin-coated onto substrates, and annealed at 500°C to form crystalline films.
Structural and Optical Properties
Mo Doping (%) | Annealing Temp. | Bandgap (eV) | Resistivity (Ω·cm) |
---|---|---|---|
0 | 500°C | 3.27 | 1.2 × 10⁻³ |
2 | 500°C | 3.18 | 8.5 × 10⁻⁴ |
5 | 500°C | 3.05 | 3.7 × 10⁻⁴ |
Mo doping reduces bandgap energy via the Burstein-Moss effect and improves electrical conductivity due to donor states from Mo⁶⁺ ions.
Mechanochemical Synthesis of ZnMoO₄
Mechanochemical synthesis offers a solvent-free route to ZnMoO₄ by ball-milling ZnO and MoO₃ powders (1:1 molar ratio) at 300 RPM for 10 hours. Post-milling calcination at 600°C for 4 hours yields phase-pure ZnMoO₄ with a scheelite structure.
Milling vs. Calcination Effects
Milling Time (h) | Calcination Temp. (°C) | Crystallite Size (nm) | Surface Area (m²/g) |
---|---|---|---|
6 | 600 | 21 | 4.8 |
10 | 600 | 35 | 3.2 |
Prolonged milling reduces crystallite size but increases surface defects, while higher calcination temperatures promote crystallite growth.
Comparative Analysis of Synthesis Methods
Method | Advantages | Limitations | Typical Applications |
---|---|---|---|
Hydrothermal | High crystallinity, morphology control | Long reaction times | Photocatalysts, sensors |
Solution-Based | Scalable, high purity | Requires pH adjustment | Corrosion inhibitors, pigments |
Sol-Gel | Uniform films, low-temperature | Shrinkage during annealing | Optoelectronics, coatings |
Mechanochemical | Solvent-free, rapid | Energy-intensive milling | Bulk ceramics, catalysts |
化学反応の分析
Types of Reactions: Molybdenum zinc oxide undergoes various chemical reactions, including oxidation, reduction, and substitution. For example, molybdenum in its +6 oxidation state can be reduced to +4 by ascorbic acid . Additionally, this compound can react with oxygen at high temperatures to form molybdenum trioxide .
Common Reagents and Conditions:
Oxidation: Oxygen (O₂) at high temperatures.
Reduction: Ascorbic acid.
Substitution: Various organic and inorganic reagents depending on the desired product.
Major Products:
Oxidation: Molybdenum trioxide (MoO₃).
Reduction: Molybdenum dioxide (MoO₂).
科学的研究の応用
Electronic Applications
Molybdenum-doped zinc oxide is widely researched for its potential use in transparent conductive oxides (TCOs). The incorporation of molybdenum enhances the electrical conductivity and optical transparency of zinc oxide thin films, making them suitable for applications in:
- Solar Cells : Mo-doped ZnO films serve as effective transparent electrodes in solar cell devices. Studies have shown that the doping process improves the film's conductivity without significantly compromising transparency, which is crucial for efficient light absorption in photovoltaic applications .
- Thin Film Transistors : The electrical properties of Mo-doped ZnO make it a promising candidate for use in thin film transistors, which are essential components in display technologies and flexible electronics. The improved charge carrier mobility due to molybdenum doping enhances the performance of these devices .
Photocatalytic Applications
Molybdenum zinc oxide exhibits excellent photocatalytic properties, making it effective for environmental remediation processes:
- Water Purification : Mo-doped ZnO has been shown to effectively degrade organic pollutants in water under UV light irradiation. The presence of molybdenum enhances the photocatalytic activity by increasing the generation of reactive oxygen species, which are crucial for breaking down contaminants .
- Antibacterial Activity : Research indicates that Mo-doped ZnO can be utilized to develop antibacterial coatings. The photocatalytic activity under UV light not only degrades organic pollutants but also kills bacteria, making it suitable for applications in healthcare and sanitation .
Structural and Optical Properties
The structural integrity and optical characteristics of molybdenum-doped zinc oxide thin films are critical for their applications:
- Thin Film Characteristics : Studies have demonstrated that varying the concentration of molybdenum affects the crystallite size and surface morphology of ZnO films. For instance, increasing molybdenum content leads to a smoother surface with enhanced optical transmittance in the visible spectrum .
- X-ray Diffraction Analysis : X-ray diffraction studies reveal that Mo-doping influences the crystal structure of ZnO, promoting a wurtzite structure that is favorable for electronic applications. The peak intensities related to Mo-based compounds increase with higher doping concentrations, indicating successful incorporation into the ZnO lattice .
Case Study 1: Photocatalytic Degradation of Dyes
A study explored the use of Mo-doped ZnO as a photocatalyst for degrading rhodamine B dye in wastewater. The results showed nearly 100% degradation efficiency within 90 minutes under UV light exposure. This highlights the effectiveness of molybdenum doping in enhancing photocatalytic activity .
Case Study 2: Transparent Conductive Oxides
Research focused on the application of Mo-doped ZnO in solar cells demonstrated that films with optimal molybdenum concentrations exhibited superior conductivity and transparency compared to undoped films. This advancement has significant implications for improving solar energy conversion efficiencies .
作用機序
The mechanism of action of molybdenum zinc oxide involves its ability to interact with various molecular targets and pathways. For instance, in photothermal therapy, molybdenum oxide nanoparticles exhibit strong localized surface plasmon resonance absorption in the near-infrared region, enabling them to ablate cancer cells . Additionally, molybdenum acts as a cofactor for enzymes such as xanthine oxidoreductase, sulfite oxidase, and aldehyde oxidase .
類似化合物との比較
Undoped Zinc Oxide (ZnO)
- Structural Differences: Pure ZnO adopts a wurtzite structure, whereas ZnMoO₄ has layered octahedral/tetrahedral coordination .
- Electrical Properties: Mo doping reduces ZnO’s resistivity from ~10⁻³–10⁻⁴ Ω·cm (pure ZnO) to 9.4×10⁻⁴ Ω·cm, with carrier concentration increasing to 3.1×10²⁰ cm⁻³ .
- Applications: ZnO is widely used in sensors and solar cells, but Mo-ZnO offers superior mechanical strength and thermoelectric efficiency, making it suitable for high-stress environments .
Indium Tin Oxide (ITO)
- Optoelectronic Performance: ITO has higher conductivity (~10⁶ S/m) but lower visible-light transmittance (~80%) compared to Mo-ZnO (168,067 S/m, 87% transmittance) .
- Cost and Sustainability: Mo-ZnO avoids indium scarcity, offering a cost-effective alternative for TCO applications .
Tungsten-Doped Molybdenum Oxide (Mo-W Oxides)
- Synthesis : Mo-W oxides are synthesized via flame methods, while ZnMoO₄ uses solution-based routes .
- Functionality : Mo-W oxides excel in catalysis and gas sensing, whereas ZnMoO₄’s luminescence (due to Mo) and photovoltaic efficiency define its niche .
Aluminum-Doped Zinc Oxide (AZO)
- Conductivity: AZO achieves ~10⁴ S/m, significantly lower than Mo-ZnO’s 1.68×10⁵ S/m .
- Stability: AZO degrades in humid environments, whereas Mo-ZnO maintains stability under mechanical stress .
Molybdenum Disulfide (MoS₂)
- Electronic Structure : MoS₂ is a semiconductor with a tunable bandgap (1.2–1.8 eV), contrasting ZnMoO₄’s wide bandgap (3.43 eV) .
- Applications: MoS₂ is used in nanoelectronics and antimicrobial coatings, while ZnMoO₄ focuses on energy storage and optoelectronics .
Data Tables
Table 1: Key Properties of ZnMoO₄ and Comparable Materials
Research Findings and Challenges
- Photovoltaics : ZnMoO₄’s high conductivity and transparency make it a viable ITO substitute, but long-term stability under UV exposure requires further study .
- Thermoelectrics: Mo doping in ZnO enhances carrier concentration but reduces mobility, necessitating band engineering for optimal performance .
- Environmental Remediation: ZnMoO₄-based nanosorbents show promise in radioactive waste adsorption (e.g., 83.65 mg/g for ⁶⁰Co) but face scalability challenges .
Q & A
Basic Research Questions
Q. What are the standard methods for synthesizing zinc molybdate (ZnMoO₄) in laboratory settings?
Zinc molybdate is typically synthesized via solid-state reactions between ZnO and MoO₃ at elevated temperatures (400–700°C). The reaction proceeds as:
Key methodological considerations include:
- Stoichiometric ratios : Precise molar ratios (1:1) of ZnO and MoO₃ are critical to avoid secondary phases like Zn₃Mo₂O₉, which forms at higher temperatures (\sim850°C) .
- Temperature control : Phase purity depends on reaction temperature. For example, ZnMoO₄ forms at 550°C, while Zn₃Mo₂O₉ emerges at 850°C .
- Characterization : X-ray diffraction (XRD) and thermal gravimetric analysis (TGA) are essential to confirm phase formation and stability .
Table 1 : Synthesis Conditions for ZnO-MoO₃ System
Compound | Temperature Range | Key Reaction Conditions |
---|---|---|
ZnMoO₄ | 400–700°C | 1:1 molar ratio, slow cooling |
Zn₃Mo₂O₉ | \sim850°C | Excess ZnO, rapid quenching |
Q. How do researchers determine the empirical formula of zinc molybdate compounds?
Empirical formulas are derived through:
- Elemental analysis : Combustion analysis or inductively coupled plasma mass spectrometry (ICP-MS) quantifies Zn and Mo content.
- Stoichiometric validation : Confirming the 1:1 Zn:Mo ratio via XRD lattice parameters and Rietveld refinement .
- Error mitigation : Replicate experiments and control atmospheric conditions (e.g., inert gas) to prevent oxidation or moisture interference .
Advanced Research Questions
Q. What experimental strategies resolve contradictions in reported phase equilibria for the MoO₃-ZnO system?
Discrepancies in phase diagrams (e.g., formation of ZnMoO₄ vs. Zn₃Mo₂O₉) arise from differences in heating rates, cooling methods, and precursor purity. To address this:
- Controlled thermal profiling : Use slow heating rates (<5°C/min) and isothermal holds to ensure equilibrium conditions .
- In-situ characterization : Synchrotron XRD or Raman spectroscopy during heating/cooling cycles captures transient phases .
- Comparative studies : Cross-validate results with thermodynamic modeling (e.g., CALPHAD) to predict stable phases under varying conditions .
Table 2 : Key Phases in MoO₃-ZnO System
Phase | Stability Range | Structural Features |
---|---|---|
ZnMoO₄ | 550–700°C | Monoclinic structure, P2₁/c space group |
Zn₃Mo₂O₉ | >800°C | Orthorhombic structure, layered Mo-O units |
Q. How can researchers optimize the synthesis of ZnMoO₄ for photocatalytic applications?
Enhanced photocatalytic activity requires:
- Defect engineering : Introduce oxygen vacancies via calcination in reducing atmospheres (e.g., H₂/N₂).
- Morphological control : Hydrothermal synthesis yields nanostructured ZnMoO₄ with higher surface area .
- Doping strategies : Incorporate transition metals (e.g., Cu²⁺) to modify bandgap energies, validated via UV-Vis diffuse reflectance spectroscopy .
Q. What methodologies assess the environmental and health risks of ZnMoO₄ nanoparticles in laboratory settings?
- Toxicity screening : Use in vitro assays (e.g., MTT tests on human cell lines) to evaluate cytotoxicity .
- Environmental persistence : Simulate aqueous stability via pH-dependent dissolution studies, monitored by ICP-MS .
- Reporting standards : Adhere to ICMJE guidelines for documenting chemical purity, synthesis protocols, and hazard data to ensure reproducibility .
Q. Methodological Best Practices
- Data contradiction analysis : When conflicting results arise (e.g., phase stability vs. synthesis temperature), systematically isolate variables (precursor source, furnace type) and use high-purity reagents (>99.9%) .
- Reproducibility : Provide detailed experimental parameters (heating rates, ambient conditions) in supplementary materials, as recommended by the Beilstein Journal of Organic Chemistry .
特性
IUPAC Name |
zinc;molybdenum;oxygen(2-) | |
---|---|---|
Source | PubChem | |
URL | https://pubchem.ncbi.nlm.nih.gov | |
Description | Data deposited in or computed by PubChem | |
InChI |
InChI=1S/Mo.4O.Zn/q;4*-2;+2 | |
Source | PubChem | |
URL | https://pubchem.ncbi.nlm.nih.gov | |
Description | Data deposited in or computed by PubChem | |
InChI Key |
CEKVBJSJDXQDQR-UHFFFAOYSA-N | |
Source | PubChem | |
URL | https://pubchem.ncbi.nlm.nih.gov | |
Description | Data deposited in or computed by PubChem | |
Canonical SMILES |
[O-2].[O-2].[O-2].[O-2].[Zn+2].[Mo] | |
Source | PubChem | |
URL | https://pubchem.ncbi.nlm.nih.gov | |
Description | Data deposited in or computed by PubChem | |
Molecular Formula |
MoO4Zn-6 | |
Source | PubChem | |
URL | https://pubchem.ncbi.nlm.nih.gov | |
Description | Data deposited in or computed by PubChem | |
DSSTOX Substance ID |
DTXSID10893960 | |
Record name | Zinc molybdate(VI) (ZnMoO4) | |
Source | EPA DSSTox | |
URL | https://comptox.epa.gov/dashboard/DTXSID10893960 | |
Description | DSSTox provides a high quality public chemistry resource for supporting improved predictive toxicology. | |
Molecular Weight |
225.3 g/mol | |
Source | PubChem | |
URL | https://pubchem.ncbi.nlm.nih.gov | |
Description | Data deposited in or computed by PubChem | |
CAS No. |
13767-32-3, 61583-60-6 | |
Record name | Zinc molybdate | |
Source | ChemIDplus | |
URL | https://pubchem.ncbi.nlm.nih.gov/substance/?source=chemidplus&sourceid=0013767323 | |
Description | ChemIDplus is a free, web search system that provides access to the structure and nomenclature authority files used for the identification of chemical substances cited in National Library of Medicine (NLM) databases, including the TOXNET system. | |
Record name | Molybdenum zinc oxide | |
Source | ChemIDplus | |
URL | https://pubchem.ncbi.nlm.nih.gov/substance/?source=chemidplus&sourceid=0061583606 | |
Description | ChemIDplus is a free, web search system that provides access to the structure and nomenclature authority files used for the identification of chemical substances cited in National Library of Medicine (NLM) databases, including the TOXNET system. | |
Record name | Molybdenum zinc oxide | |
Source | EPA Chemicals under the TSCA | |
URL | https://www.epa.gov/chemicals-under-tsca | |
Description | EPA Chemicals under the Toxic Substances Control Act (TSCA) collection contains information on chemicals and their regulations under TSCA, including non-confidential content from the TSCA Chemical Substance Inventory and Chemical Data Reporting. | |
Record name | Zinc molybdate(VI) (ZnMoO4) | |
Source | EPA DSSTox | |
URL | https://comptox.epa.gov/dashboard/DTXSID10893960 | |
Description | DSSTox provides a high quality public chemistry resource for supporting improved predictive toxicology. | |
Record name | Molybdenum zinc oxide | |
Source | European Chemicals Agency (ECHA) | |
URL | https://echa.europa.eu/substance-information/-/substanceinfo/100.057.126 | |
Description | The European Chemicals Agency (ECHA) is an agency of the European Union which is the driving force among regulatory authorities in implementing the EU's groundbreaking chemicals legislation for the benefit of human health and the environment as well as for innovation and competitiveness. | |
Explanation | Use of the information, documents and data from the ECHA website is subject to the terms and conditions of this Legal Notice, and subject to other binding limitations provided for under applicable law, the information, documents and data made available on the ECHA website may be reproduced, distributed and/or used, totally or in part, for non-commercial purposes provided that ECHA is acknowledged as the source: "Source: European Chemicals Agency, http://echa.europa.eu/". Such acknowledgement must be included in each copy of the material. ECHA permits and encourages organisations and individuals to create links to the ECHA website under the following cumulative conditions: Links can only be made to webpages that provide a link to the Legal Notice page. | |
試験管内研究製品の免責事項と情報
BenchChemで提示されるすべての記事および製品情報は、情報提供を目的としています。BenchChemで購入可能な製品は、生体外研究のために特別に設計されています。生体外研究は、ラテン語の "in glass" に由来し、生物体の外で行われる実験を指します。これらの製品は医薬品または薬として分類されておらず、FDAから任何の医療状態、病気、または疾患の予防、治療、または治癒のために承認されていません。これらの製品を人間または動物に体内に導入する形態は、法律により厳格に禁止されています。これらのガイドラインに従うことは、研究と実験において法的および倫理的な基準の遵守を確実にするために重要です。