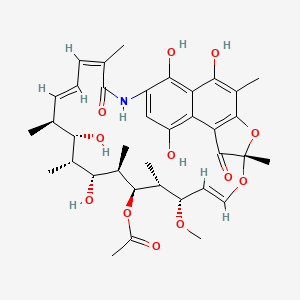
リファマイシン
概要
説明
リファマイシンは、細菌Amycolatopsis rifamycinicaによって自然に合成されるか、または人工的に合成される抗生物質のグループです。 これらは、より大きなアンサマイシンファミリーに属し、特にマイコバクテリアに対して効果的です。 リファマイシンは、結核、ハンセン病、および鳥型マイコバクテリア複合感染などの疾患の治療に使用されます 。 この化合物は、1957年にStreptomyces mediterraneiの発酵培養物から初めて単離されました .
科学的研究の応用
Rifamycin has a wide range of scientific research applications:
Chemistry: Used as a model compound for studying antibiotic synthesis and mechanisms.
Biology: Studied for its effects on bacterial RNA polymerase and its role in inhibiting bacterial growth.
Medicine: Widely used in the treatment of tuberculosis, leprosy, and other bacterial infections.
Industry: Employed in the production of various rifamycin derivatives for pharmaceutical use.
作用機序
リファマイシンは、細菌のDNA依存性RNAポリメラーゼを阻害することによって抗菌効果を発揮します。 この阻害によりRNAの合成が阻害され、細菌が死滅します。 この化合物はRNAポリメラーゼに強く結合し、RNA鎖の伸長をブロックします 。 このメカニズムは、原核生物のRNAポリメラーゼに非常に特異的であるため、リファマイシンはさまざまな細菌に対して効果的です .
類似化合物:
- リファンピシン
- リファブチン
- リファペンチン
- リファキシミン
比較: リファマイシンとその誘導体は共通の作用機序を共有していますが、薬物動態特性と臨床的用途が異なります。 たとえば、リファンピシンは結核治療に広く使用されていますが、リファキシミンは旅行者下痢の治療に使用されます 。 リファブチンとリファペンチンは吸収速度が異なり、特定の細菌感染症に使用されます 。 リファマイシンの独特の構造により、その有効性を高め、耐性を減らす改変が可能になります .
リファマイシンは、広域スペクトル活性と、他の抗生物質との交差耐性なしに細菌RNAポリメラーゼを阻害する能力により際立っています .
生化学分析
Biochemical Properties
Rifamycin plays a crucial role in biochemical reactions by inhibiting bacterial DNA-dependent RNA polymerase. This inhibition prevents the transcription of bacterial DNA into RNA, effectively halting protein synthesis and leading to bacterial cell death . Rifamycin interacts with several biomolecules, including enzymes and proteins. It binds specifically to the beta subunit of RNA polymerase, forming a stable complex that obstructs the elongation of the RNA chain . This interaction is highly selective, ensuring that rifamycin targets bacterial cells without affecting human cells.
Cellular Effects
Rifamycin exerts significant effects on various types of cells and cellular processes. In bacterial cells, it rapidly kills fast-dividing strains as well as “persister” cells, which remain dormant and evade antibiotic activity . This dual action makes rifamycin particularly effective against chronic infections. Additionally, rifamycin influences cell signaling pathways and gene expression by inhibiting RNA synthesis, leading to a cascade of effects that disrupt cellular metabolism and function .
Molecular Mechanism
At the molecular level, rifamycin’s mechanism of action involves binding to the DNA-dependent RNA polymerase of prokaryotes. This binding inhibits the enzyme’s activity, preventing the transcription of DNA into RNA . The inhibition is achieved through the formation of a stable complex between rifamycin and the beta subunit of RNA polymerase, which blocks the elongation of the RNA chain . This selective inhibition ensures that rifamycin effectively targets bacterial cells while minimizing effects on human cells.
Temporal Effects in Laboratory Settings
In laboratory settings, the effects of rifamycin have been observed to change over time. Rifamycin is known for its stability and sustained activity, making it effective in long-term treatments . Resistance can develop over time due to mutations in the RNA polymerase gene, which reduce the binding affinity of rifamycin . Studies have shown that rifamycin maintains its antibacterial activity for extended periods, but its effectiveness can diminish with prolonged use due to the emergence of resistant bacterial strains .
Dosage Effects in Animal Models
The effects of rifamycin vary with different dosages in animal models. At therapeutic doses, rifamycin effectively eliminates bacterial infections without causing significant adverse effects . At high doses, rifamycin can cause toxic effects, including hepatotoxicity and gastrointestinal disturbances . Studies in animal models have shown that the therapeutic window for rifamycin is relatively wide, allowing for effective treatment with minimal risk of toxicity .
Metabolic Pathways
Rifamycin is metabolized primarily in the liver, where it undergoes biotransformation to several active metabolites . These metabolites are excreted mainly in bile and, to a lesser extent, in urine . Rifamycin induces the cytochrome P450 enzyme system, particularly CYP3A4, which can lead to interactions with other drugs metabolized by this pathway . This induction can affect the metabolic flux and levels of various metabolites, influencing the overall pharmacokinetics of rifamycin .
Transport and Distribution
Rifamycin is well-absorbed orally and widely distributed in body tissues and fluids, including cerebrospinal fluid . It is concentrated in polymorphonuclear granulocytes and macrophages, facilitating the clearance of bacteria from abscesses . Rifamycin is transported within cells by binding to plasma proteins, which aids in its distribution to various tissues . Its high lipid solubility allows it to penetrate cell membranes and reach intracellular targets effectively .
Subcellular Localization
Rifamycin’s subcellular localization is primarily within the cytoplasm, where it exerts its antibacterial effects by targeting RNA polymerase . It does not require specific targeting signals or post-translational modifications to reach its site of action. Instead, its high affinity for bacterial RNA polymerase ensures that it localizes to the appropriate subcellular compartment to inhibit RNA synthesis .
準備方法
合成経路と反応条件: リファマイシンは、さまざまな方法で合成することができます。 一般的な方法の1つは、マイクロリアクター内でリファマイシンSをtert-ブチルアミンと反応させることであり、リファンピシンが全体の収率67%で生成されます 。 別の方法には、リファマイシンナトリウム注射剤の調製が含まれ、注射用水に原料を溶解し、pHを調整し、溶液を濾過するなどのステップが含まれます .
工業的生産方法: リファマイシンの工業的生産には、しばしばAmycolatopsis rifamycinicaを用いた発酵プロセスが含まれます。 発酵培養物は、リファマイシンの収率を最適化するために注意深く監視および制御されます。 コスト削減と効率向上のため、連続フロー合成法も研究されています .
化学反応の分析
反応の種類: リファマイシンは、酸化、還元、置換などのさまざまな化学反応を受けます。 たとえば、リファマイシンSVからリファンピシンへの変換には、1-アミノ-4-メチルピペラジンとの反応が含まれます .
一般的な試薬と条件: リファマイシン誘導体の合成に使用される一般的な試薬には、tert-ブチルアミン、1-アミノ-4-メチルピペラジン、およびメタ重亜硫酸ナトリウムが含まれます。 反応条件には、しばしば制御されたpH、温度、および連続フロー合成のためのマイクロリアクターの使用が含まれます .
主な生成物: これらの反応から生成される主な生成物には、リファンピシン、リファブチン、リファペンチン、およびリファキシミンが含まれます。 これらの誘導体は、物理化学的性質にわずかな違いがありますが、同じコア構造を共有しています .
4. 科学研究への応用
リファマイシンは、幅広い科学研究に適用されています。
類似化合物との比較
- Rifampicin
- Rifabutin
- Rifapentine
- Rifaximin
Comparison: Rifamycin and its derivatives share a common mechanism of action but differ in their pharmacokinetic properties and clinical uses. For example, rifampicin is widely used for tuberculosis treatment, while rifaximin is used for treating traveler’s diarrhea . Rifabutin and rifapentine have different absorption rates and are used for specific bacterial infections . The unique structure of rifamycin allows for modifications that enhance its efficacy and reduce resistance .
Rifamycin stands out due to its broad-spectrum activity and its ability to inhibit bacterial RNA polymerase without cross-resistance with other antibiotics .
特性
Rifamycins, as well as all the other members of this group, present an antibacterial mechanism of action related to the inhibition of RNA synthesis. This mechanism of action is done by the strong binding to the DNA-dependent RNA polymerase of prokaryotes. The inhibition of the RNA synthesis is thought to be related with the initiation phase of the process and to involve stacking interactions between the naphthalene ring and the aromatic moiety in the polymerase. As well, it has been suggested that the presence of zinc atoms in the polymerase allows for the binding of phenolic -OH groups of the naphthalene ring. In eukaryotic cells, the binding is significantly reduced making them at least 100 to 10,000 times less sensitive to the action of rifamycins. The members of the rifamycin family present the same mechanism of action and the structural modifications are usually related to pharmacokinetic properties as well as to the interaction with eukaryotic cells. | |
CAS番号 |
6998-60-3 |
分子式 |
C37H47NO12 |
分子量 |
697.8 g/mol |
IUPAC名 |
[(7S,11S,12R,13S,14R,15R,16R,17S,18S,19Z,21Z)-2,15,17,27,29-pentahydroxy-11-methoxy-3,7,12,14,16,18,22-heptamethyl-6,23-dioxo-8,30-dioxa-24-azatetracyclo[23.3.1.14,7.05,28]triaconta-1(29),2,4,9,19,21,25,27-octaen-13-yl] acetate |
InChI |
InChI=1S/C37H47NO12/c1-16-11-10-12-17(2)36(46)38-23-15-24(40)26-27(32(23)44)31(43)21(6)34-28(26)35(45)37(8,50-34)48-14-13-25(47-9)18(3)33(49-22(7)39)20(5)30(42)19(4)29(16)41/h10-16,18-20,25,29-30,33,40-44H,1-9H3,(H,38,46)/b11-10-,14-13?,17-12-/t16-,18+,19+,20+,25-,29-,30+,33+,37-/m0/s1 |
InChIキー |
HJYYPODYNSCCOU-PTWHBISLSA-N |
異性体SMILES |
C[C@H]1/C=C\C=C(/C(=O)NC2=CC(=C3C(=C2O)C(=C(C4=C3C(=O)[C@](O4)(OC=C[C@@H]([C@H]([C@H]([C@@H]([C@@H]([C@@H]([C@H]1O)C)O)C)OC(=O)C)C)OC)C)C)O)O)\C |
SMILES |
CC1C=CC=C(C(=O)NC2=CC(=C3C(=C2O)C(=C(C4=C3C(=O)C(O4)(OC=CC(C(C(C(C(C(C1O)C)O)C)OC(=O)C)C)OC)C)C)O)O)C |
正規SMILES |
CC1C=CC=C(C(=O)NC2=CC(=C3C(=C2O)C(=C(C4=C3C(=O)C(O4)(OC=CC(C(C(C(C(C(C1O)C)O)C)OC(=O)C)C)OC)C)C)O)O)C |
外観 |
Solid powder |
沸点 |
972.8 ºC at 760 mm Hg |
melting_point |
171 ºC 300.0 °C |
6998-60-3 | |
純度 |
>98% (or refer to the Certificate of Analysis) |
関連するCAS |
14897-39-3 (unspecified hydrochloride salt) 15105-92-7 (mono-hydrochloride salt) 6998-60-3 (Parent) |
賞味期限 |
>3 years if stored properly |
溶解性 |
Insoluble |
保存方法 |
Dry, dark and at 0 - 4 C for short term (days to weeks) or -20 C for long term (months to years). |
同義語 |
5,6,9,17,19,21-hexahydroxy-23-methoxy-2,4,12,16,18,20,22-heptamethyl-2,7-(epoxypentadeca(1,11,13)trienimino)naphtho(2,1-b)furan-1,11(2-H)-dione, 21-acetate Aemcolo Otafa R-75-1 Rifacin Rifamicina Colirio rifamycin SV rifamycin SV, monosodium salt rifamycin SV, sodium salt Rifamycine Chibret Rifocina Rifocyna |
製品の起源 |
United States |
Retrosynthesis Analysis
AI-Powered Synthesis Planning: Our tool employs the Template_relevance Pistachio, Template_relevance Bkms_metabolic, Template_relevance Pistachio_ringbreaker, Template_relevance Reaxys, Template_relevance Reaxys_biocatalysis model, leveraging a vast database of chemical reactions to predict feasible synthetic routes.
One-Step Synthesis Focus: Specifically designed for one-step synthesis, it provides concise and direct routes for your target compounds, streamlining the synthesis process.
Accurate Predictions: Utilizing the extensive PISTACHIO, BKMS_METABOLIC, PISTACHIO_RINGBREAKER, REAXYS, REAXYS_BIOCATALYSIS database, our tool offers high-accuracy predictions, reflecting the latest in chemical research and data.
Strategy Settings
Precursor scoring | Relevance Heuristic |
---|---|
Min. plausibility | 0.01 |
Model | Template_relevance |
Template Set | Pistachio/Bkms_metabolic/Pistachio_ringbreaker/Reaxys/Reaxys_biocatalysis |
Top-N result to add to graph | 6 |
Feasible Synthetic Routes
Q1: How do rifamycins exert their antibacterial activity?
A1: Rifamycins specifically bind to the β-subunit of bacterial DNA-dependent RNA polymerase (RNAP) [, , , ]. This binding inhibits the initiation of RNA synthesis, effectively halting bacterial growth and leading to cell death [, , ].
Q2: Can rifamycins inhibit RNAP in organisms other than bacteria?
A2: While highly effective against bacterial RNAP, rifamycins demonstrate limited activity against mammalian RNAP, contributing to their selective toxicity []. There are reports of rifamycins inhibiting the reverse transcriptase (RT) of some RNA viruses [].
Q3: What are the essential structural features of rifamycins responsible for their activity?
A3: Rifamycins require a naphthalene ring with oxygen atoms at specific positions, two unsubstituted hydroxyl groups on the ansa chain, and a precise spatial arrangement of oxygen atoms for optimal activity []. The ansa bridge links non-adjacent positions of the aromatic nucleus [].
Q4: Which structural modifications of rifamycins have been explored to enhance their activity?
A4: Most modifications focus on the C-3 or C-4 positions of the naphthoquinone/naphthohydroquinone chromophore, leading to derivatives like rifampicin []. One example is the addition of a benzoxazine ring, yielding benzoxazinorifamycins like rifalazil, which exhibit improved activity against rifamycin-resistant strains [, , ].
Q5: How do bacteria develop resistance to rifamycins?
A5: The most common mechanism involves mutations in the rifamycin resistance-determining region (RRDR) within the rpoB gene encoding the β-subunit of RNAP [, ]. These mutations alter the drug's binding site, reducing its efficacy [, ].
Q6: Are there enzymes that contribute to rifamycin resistance?
A6: Yes, some bacteria possess enzymes that inactivate rifamycins. One example is the ADP-ribosyltransferase Arr, found in Mycobacterium abscessus, which adds ADP-ribose to rifampicin, rendering it ineffective [, ]. Another mechanism involves enzymatic degradation of rifamycins by Rox enzymes, a type of flavin monooxygenase [].
Q7: Is there cross-resistance between rifamycins and other antibiotic classes?
A8: Fidaxomicin, another RNAP inhibitor, demonstrates a lower propensity for the development of resistance to rifamycins and exhibits no cross-resistance with rifamycins, highlighting differences in their mechanisms of action [].
Q8: How is the rifamycin scaffold biosynthesized?
A9: The core structure is assembled by a type I modular polyketide synthase (PKS) using 3-amino-5-hydroxybenzoic acid (AHBA), acetate, and propionate as building blocks []. The polyketide framework of rifamycin B is assembled from 3-amino-5-hydroxybenzoic acid (AHBA), two molecules of acetate, and eight molecules of propionate [].
Q9: What are some of the key intermediates in the rifamycin biosynthetic pathway?
A10: Proansamycin X is a proposed early macrocyclic intermediate []. Rifamycin W is a predominant intermediate that undergoes significant structural rearrangements to form rifamycin B [, ]. Studies on mutants with inactivated rifF gene provide insights into the timing of naphthoquinone ring formation and other biosynthetic steps [].
Q10: What is the role of rifamycin oxidase in rifamycin production?
A11: Rifamycin oxidase catalyzes the biotransformation of the less active rifamycin B to the more potent rifamycin S, a key intermediate for synthesizing various semi-synthetic rifamycins [, ].
Q11: How can the production of rifamycin B be improved?
A11: Several approaches have been explored, including:
- Strain Optimization: Selecting for high-yielding strains based on colony morphology and implementing genetic modifications, such as gene amplification [, ].
- Media Optimization: Replacing ammonium sulfate with potassium nitrate or ammonium nitrate as the nitrogen source can significantly enhance production [, ].
- Feeding Strategies: Implementing a fed-batch fermentation process with optimized feeding of glucose, yeast extract, and nitrogen sources can further improve rifamycin B yields [].
Q12: What are the clinical applications of rifamycins?
A12: Rifamycins are crucial for treating infections caused by:
- Mycobacterium tuberculosis (tuberculosis) [, , , , ]
- Mycobacterium leprae (leprosy) []
- AIDS-related mycobacterial infections [, , ]
- Staphylococcus aureus infections [, , ]
- Streptococcus pyogenes infections []
Q13: How do rifamycins behave within the body?
A14: The pharmacokinetic properties of rifamycins are complex, leading to significant inter-individual variability in drug exposure []. Factors like body weight, disease state, food intake, and genetic polymorphisms in drug transporter proteins can influence their absorption, distribution, metabolism, and excretion [].
Q14: What is the current research focus on improving rifamycin therapy?
A14: Current research focuses on:
- Optimizing Dosing: Exploring higher doses to shorten TB treatment duration and investigating the relationship between rifamycin dose and toxicity [].
- Developing New Analogs: Creating derivatives with enhanced activity against resistant strains, improved pharmacokinetic properties, and reduced toxicity [, , , ].
- Exploring Drug Synergies: Investigating rifamycin combinations with other antibiotics or host-directed therapies to enhance efficacy and combat resistance [].
試験管内研究製品の免責事項と情報
BenchChemで提示されるすべての記事および製品情報は、情報提供を目的としています。BenchChemで購入可能な製品は、生体外研究のために特別に設計されています。生体外研究は、ラテン語の "in glass" に由来し、生物体の外で行われる実験を指します。これらの製品は医薬品または薬として分類されておらず、FDAから任何の医療状態、病気、または疾患の予防、治療、または治癒のために承認されていません。これらの製品を人間または動物に体内に導入する形態は、法律により厳格に禁止されています。これらのガイドラインに従うことは、研究と実験において法的および倫理的な基準の遵守を確実にするために重要です。