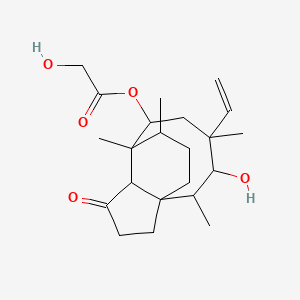
プレウロムチリン
概要
説明
Pleuromutilin is a natural antibiotic first discovered in the 1950s from an edible mushroom—Pleurotus mutilus. The pleuromutilins are semisynthetic derivatives of the parent compound that have been used in veterinary medicine for over 3 decades with little evidence of resistant development .
Synthesis Analysis
Pleuromutilin is an antibiotic diterpenoid made by Clitopilus passeckerianus and related fungi. It is the progenitor of a growing class of semi-synthetic antibiotics used in veterinary and human medicine . A linear pathway for pleuromutilin biosynthesis was established, and two shunt pathways involving Pl-sdr and Pl-atf were identified through the rational heterologous expression of combinations of pleuromutilin biosynthetic genes in Aspergillus oryzae .
Molecular Structure Analysis
Pleuromutilin is a diterpenoid antimicrobial isolated from cultures of Clitopilus passeckerianus and related basidiomycete fungi . It has a unique induced-fit type of action that closes the binding pocket within a ribosome, conferring close contact of the drug to its target, therefore improving therapeutic efficacy .
Chemical Reactions Analysis
The biosynthesis of pleuromutilin involves various chemical reactions. It was observed that the absence of various functional groups – 3 ketone, 11 hydroxyl group or 21 ketone – from the pleuromutilin framework affected the antibacterial activity of pleuromutilin congeners .
Physical And Chemical Properties Analysis
Pleuromutilin is a diterpenoid antimicrobial isolated from cultures of Clitopilus passeckerianus and related basidiomycete fungi . Its physical and chemical properties are influenced by its molecular structure and the presence or absence of various functional groups .
科学的研究の応用
抗菌剤
プレウロムチリンとその誘導体は、抗菌作用について広く研究されています . それらは、細菌リボソームの50Sサブユニットと相互作用して細菌タンパク質合成を阻害することにより、細菌およびマイコプラズマに対して優れた抗菌活性を示します . プレウロムチリン化合物は哺乳類のリボソームに結合しないため、抗生物質開発の有望な候補となっています .
メチシリン耐性黄色ブドウ球菌 (MRSA) の治療
プレウロムチリン誘導体は、MRSAの治療において大きな可能性を示しています . たとえば、化合物1は、黄色ブドウ球菌ATCC 29213(S. aureus)およびMRSAの両方に対して最も高い抗菌活性を示し、最小発育阻止濃度(MIC)は< 0.0625 μg/mLでした .
定量的構造活性相関 (QSAR) モデルを使用した薬物最適化
QSAR法は、新規薬物リードのバーチャルスクリーニングとプレウロムチリンの最適化に使用されてきました . 得られたQSARモデルに基づいて、チオール官能基化側鎖を持つプレウロムチリン化合物が設計および合成されました .
獣医用抗生物質
プレウロムチリン誘導体であるチアミュリンとバルネムリンは、獣医用抗生物質として成功裏に開発されています . それらは、その独特の作用機序により、ますます科学的な注目を集めています .
グラム陽性病原体の治療
プレウロムチリン抗生物質は、グラム陽性病原体に対して抗菌活性を示します . それらはまた、いくつかの偏性嫌気性グラム陰性細菌を網羅しています . グラム陽性細菌が現在承認されている抗生物質に対する耐性を高めるにつれて、プレウロムチリン抗生物質は、ヒトで使用される全身性抗菌薬を開発するために調査されました .
新規誘導体の合成
新規プレウロムチリン誘導体が、黄色ブドウ球菌 (S. aureus) に対する阻害剤として合成されています. ピラゾール環のN-1位に多様な置換基が導入されました . これらの半合成誘導体は、in vitro抗菌活性について評価されました .
作用機序
Target of Action
Pleuromutilin and its derivatives are antibacterial drugs that primarily target the peptidyl transferase component of the 50S subunit of ribosomes . This component plays a crucial role in protein synthesis in bacteria .
Mode of Action
Pleuromutilin inhibits bacterial protein synthesis by binding to the peptidyl transferase center of the 50S ribosomal subunit . This interaction prevents the binding of transfer RNA for peptide transfer , thereby inhibiting peptide bond formation . The pleuromutilin core interacts with the ribosomal nucleotides mainly through hydrophobic interactions, van der Waal forces, and hydrogen bonds with nucleotides of domain V of 23S rRNA .
Biochemical Pathways
Pleuromutilin belongs to the class of secondary metabolites known as terpenes, which are produced in fungi through the mevalonate pathway . Its synthetic bottleneck lies in the production of the precursor GGPP and the subsequent formation of the tricyclic structure, which is catalyzed by Pl-cyc, a bifunctional diterpene synthase . Two shunt pathways involving Pl-sdr and Pl-atf have also been identified .
Pharmacokinetics
It exhibits promising pharmacokinetic properties, including a half-life of 3.37 hours, a clearance rate of 0.35 L/h/kg, and an area under the plasma concentration-time curve (AUC) of 70.68 μg h/mL . These properties impact the bioavailability of the drug and its therapeutic efficacy.
Result of Action
The result of pleuromutilin’s action is the inhibition of bacterial protein synthesis, leading to the death of the bacteria . Pleuromutilin is active against typical and atypical bacteria that cause community-acquired pneumonia . It is also active against sexually transmitted pathogens and may have a role in the treatment of drug-resistant strains of N. gonorrhoeae and M. genitalium .
Action Environment
The action of pleuromutilin can be influenced by environmental factors. For instance, the tissue distribution of DPTM, a pleuromutilin derivative, showed that it was diffused into all tested tissues, especially into the intestine and lung . This suggests that the drug’s action, efficacy, and stability can be influenced by the environment in which it is administered.
Safety and Hazards
将来の方向性
Given the rise of antibiotic-resistant organisms, the use of pleuromutilin may soon change. It may provide a valuable intravenous/oral monotherapy alternative to fluoroquinolones or macrolides for empiric treatment of patients with CABP, including cases of patients at risk for poor outcomes due to age or various comorbidities .
生化学分析
Biochemical Properties
Pleuromutilin plays a crucial role in biochemical reactions by inhibiting bacterial protein synthesis. It achieves this by binding to the peptidyl transferase center of the bacterial ribosome, specifically the 50S subunit . This binding prevents the proper alignment of transfer RNA (tRNA) and messenger RNA (mRNA), thereby inhibiting peptide bond formation and protein synthesis. The interaction of pleuromutilin with the ribosome is highly selective, making it effective against bacterial cells while having minimal impact on eukaryotic cells .
Cellular Effects
Pleuromutilin exerts significant effects on various types of cells and cellular processes. In bacterial cells, it disrupts protein synthesis, leading to cell death. This disruption affects cell signaling pathways, gene expression, and cellular metabolism. By inhibiting protein synthesis, pleuromutilin prevents the bacteria from producing essential proteins required for their growth and survival . This action makes pleuromutilin an effective antibiotic against a wide range of bacterial pathogens.
Molecular Mechanism
At the molecular level, pleuromutilin exerts its effects by binding to the peptidyl transferase center of the bacterial ribosome. This binding involves interactions with specific nucleotides within the ribosomal RNA, leading to the inhibition of peptide bond formation . The tricyclic core of pleuromutilin and its C14 side chain play a crucial role in its binding affinity and specificity. By preventing the proper alignment of tRNA and mRNA, pleuromutilin effectively halts protein synthesis, leading to bacterial cell death .
Temporal Effects in Laboratory Settings
In laboratory settings, the effects of pleuromutilin have been observed to change over time. The stability and degradation of pleuromutilin can influence its long-term effects on cellular function. Studies have shown that pleuromutilin remains stable under various conditions, allowing for sustained antibacterial activity . Prolonged exposure to pleuromutilin can lead to the development of bacterial resistance, which may reduce its effectiveness over time .
Dosage Effects in Animal Models
The effects of pleuromutilin vary with different dosages in animal models. At therapeutic doses, pleuromutilin effectively inhibits bacterial growth without causing significant adverse effects . At higher doses, pleuromutilin can exhibit toxic effects, including hepatotoxicity and nephrotoxicity . These dosage-dependent effects highlight the importance of optimizing the dosage to achieve the desired therapeutic outcomes while minimizing potential toxicity.
Metabolic Pathways
Pleuromutilin is involved in various metabolic pathways, including its biosynthesis and degradation. The major metabolic pathway that deactivates pleuromutilin involves cytochrome P450 oxidation at specific positions within the molecule . This oxidation leads to the formation of less active metabolites, which are subsequently excreted from the body. The involvement of cytochrome P450 enzymes in pleuromutilin metabolism underscores the importance of considering potential drug interactions when using pleuromutilin in clinical settings .
Transport and Distribution
Within cells and tissues, pleuromutilin is transported and distributed through various mechanisms. It interacts with specific transporters and binding proteins that facilitate its uptake and distribution . Pleuromutilin has been shown to accumulate in certain tissues, such as the lungs and intestines, where it exerts its antibacterial effects . The distribution of pleuromutilin within the body is influenced by factors such as tissue permeability and blood flow .
Subcellular Localization
The subcellular localization of pleuromutilin is primarily within the bacterial ribosome, where it exerts its inhibitory effects on protein synthesis . The targeting of pleuromutilin to the ribosome is facilitated by specific binding interactions with ribosomal RNA and proteins. These interactions ensure that pleuromutilin reaches its site of action and effectively inhibits bacterial protein synthesis .
特性
IUPAC Name |
(4-ethenyl-3-hydroxy-2,4,7,14-tetramethyl-9-oxo-6-tricyclo[5.4.3.01,8]tetradecanyl) 2-hydroxyacetate | |
---|---|---|
Details | Computed by Lexichem TK 2.7.0 (PubChem release 2021.05.07) | |
Source | PubChem | |
URL | https://pubchem.ncbi.nlm.nih.gov | |
Description | Data deposited in or computed by PubChem | |
InChI |
InChI=1S/C22H34O5/c1-6-20(4)11-16(27-17(25)12-23)21(5)13(2)7-9-22(14(3)19(20)26)10-8-15(24)18(21)22/h6,13-14,16,18-19,23,26H,1,7-12H2,2-5H3 | |
Details | Computed by InChI 1.0.6 (PubChem release 2021.05.07) | |
Source | PubChem | |
URL | https://pubchem.ncbi.nlm.nih.gov | |
Description | Data deposited in or computed by PubChem | |
InChI Key |
ZRZNJUXESFHSIO-UHFFFAOYSA-N | |
Details | Computed by InChI 1.0.6 (PubChem release 2021.05.07) | |
Source | PubChem | |
URL | https://pubchem.ncbi.nlm.nih.gov | |
Description | Data deposited in or computed by PubChem | |
Canonical SMILES |
CC1CCC23CCC(=O)C2C1(C(CC(C(C3C)O)(C)C=C)OC(=O)CO)C | |
Details | Computed by OEChem 2.3.0 (PubChem release 2021.05.07) | |
Source | PubChem | |
URL | https://pubchem.ncbi.nlm.nih.gov | |
Description | Data deposited in or computed by PubChem | |
Molecular Formula |
C22H34O5 | |
Details | Computed by PubChem 2.1 (PubChem release 2021.05.07) | |
Source | PubChem | |
URL | https://pubchem.ncbi.nlm.nih.gov | |
Description | Data deposited in or computed by PubChem | |
Molecular Weight |
378.5 g/mol | |
Details | Computed by PubChem 2.1 (PubChem release 2021.05.07) | |
Source | PubChem | |
URL | https://pubchem.ncbi.nlm.nih.gov | |
Description | Data deposited in or computed by PubChem | |
CAS RN |
125-65-5 | |
Record name | Pleuromulin | |
Source | European Chemicals Agency (ECHA) | |
URL | https://echa.europa.eu/substance-information/-/substanceinfo/100.004.316 | |
Description | The European Chemicals Agency (ECHA) is an agency of the European Union which is the driving force among regulatory authorities in implementing the EU's groundbreaking chemicals legislation for the benefit of human health and the environment as well as for innovation and competitiveness. | |
Explanation | Use of the information, documents and data from the ECHA website is subject to the terms and conditions of this Legal Notice, and subject to other binding limitations provided for under applicable law, the information, documents and data made available on the ECHA website may be reproduced, distributed and/or used, totally or in part, for non-commercial purposes provided that ECHA is acknowledged as the source: "Source: European Chemicals Agency, http://echa.europa.eu/". Such acknowledgement must be included in each copy of the material. ECHA permits and encourages organisations and individuals to create links to the ECHA website under the following cumulative conditions: Links can only be made to webpages that provide a link to the Legal Notice page. | |
Retrosynthesis Analysis
AI-Powered Synthesis Planning: Our tool employs the Template_relevance Pistachio, Template_relevance Bkms_metabolic, Template_relevance Pistachio_ringbreaker, Template_relevance Reaxys, Template_relevance Reaxys_biocatalysis model, leveraging a vast database of chemical reactions to predict feasible synthetic routes.
One-Step Synthesis Focus: Specifically designed for one-step synthesis, it provides concise and direct routes for your target compounds, streamlining the synthesis process.
Accurate Predictions: Utilizing the extensive PISTACHIO, BKMS_METABOLIC, PISTACHIO_RINGBREAKER, REAXYS, REAXYS_BIOCATALYSIS database, our tool offers high-accuracy predictions, reflecting the latest in chemical research and data.
Strategy Settings
Precursor scoring | Relevance Heuristic |
---|---|
Min. plausibility | 0.01 |
Model | Template_relevance |
Template Set | Pistachio/Bkms_metabolic/Pistachio_ringbreaker/Reaxys/Reaxys_biocatalysis |
Top-N result to add to graph | 6 |
Feasible Synthetic Routes
試験管内研究製品の免責事項と情報
BenchChemで提示されるすべての記事および製品情報は、情報提供を目的としています。BenchChemで購入可能な製品は、生体外研究のために特別に設計されています。生体外研究は、ラテン語の "in glass" に由来し、生物体の外で行われる実験を指します。これらの製品は医薬品または薬として分類されておらず、FDAから任何の医療状態、病気、または疾患の予防、治療、または治癒のために承認されていません。これらの製品を人間または動物に体内に導入する形態は、法律により厳格に禁止されています。これらのガイドラインに従うことは、研究と実験において法的および倫理的な基準の遵守を確実にするために重要です。