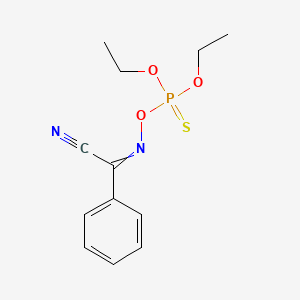
Phoxim
概要
説明
ホキシムは、O,O-ジエチル-α-シアノベンゾオキシムホスホロチオアートとしても知られており、広域スペクトル有機リン系殺虫剤です。その高い効率性と低毒性から、農業や獣医学において広く使用されています。 ホキシムは、ダニ、シラミ、その他の体外寄生虫など、さまざまな害虫に対して特に効果的です .
製造方法
合成経路と反応条件: ホキシムは通常、メタノールまたはエタノールを出発原料としてα-シアノベンズアルデヒドオキシムナトリウムを生成することにより合成されます。 この中間体は次に、ジエチルクロロチオホスフェートと反応させてホキシムを生成します . ホキシムのグリーンな製造方法では、ベンジルアルコールを出発原料として使用し、安全性の向上と廃棄物の削減などの利点があります .
工業的製造方法: 工業的な設定では、ホキシムは、亜硝酸エチルとフェニルアセトニトリルを反応させて2-シアノベンゾオキシムナトリウムを生成することによって製造されます。 この中間体は次に、トリクロロチオホスフェートとエタノールと反応させてホキシムを合成します .
作用機序
ホキシムは、神経系におけるアセチルコリンの分解を担う酵素であるアセチルコリンエステラーゼを阻害することにより作用します。 この阻害は、アセチルコリンの蓄積を引き起こし、継続的な神経インパルスを生じさせ、最終的には標的害虫の麻痺と死をもたらします . ホキシムの分子標的は、アセチルコリンエステラーゼや神経シグナル伝達に関与するその他の酵素などがあります .
類似化合物の比較
ホキシムは、クロルピリホスやピリダベンなどの他の有機リン系殺虫剤と似ています。 ホキシムは、その高い効率性と低毒性で独特であり、農業や獣医学の用途で好まれる選択肢です . テブフェノザイドやマラチオンなどの他の類似化合物も、有機リン系殺虫剤に属しています .
参考文献
生化学分析
Biochemical Properties
Phoxim plays a significant role in biochemical reactions by interacting with various enzymes and proteins. It primarily inhibits acetylcholinesterase, an enzyme crucial for breaking down acetylcholine in the nervous system. This inhibition leads to the accumulation of acetylcholine, causing continuous nerve impulse transmission . Additionally, this compound has been shown to induce oxidative stress by generating reactive oxygen species (ROS) and affecting antioxidant enzymes such as superoxide dismutase (SOD) and catalase (CAT) .
Cellular Effects
This compound affects various types of cells and cellular processes. It induces oxidative stress, leading to cellular damage and apoptosis. In renal cells, this compound exposure results in mitochondrial dysfunction, increased ROS production, and activation of apoptotic pathways . This compound also influences cell signaling pathways, gene expression, and cellular metabolism. For instance, it alters the expression of genes related to antioxidation, cell apoptosis, immunity, and the endocrine system .
Molecular Mechanism
At the molecular level, this compound exerts its effects through several mechanisms. It binds to the active site of acetylcholinesterase, inhibiting its activity and leading to the accumulation of acetylcholine . This compound also induces oxidative stress by generating ROS, which can damage cellular components such as lipids, proteins, and DNA. This oxidative stress triggers mitochondrial apoptosis, characterized by the release of cytochrome c and activation of caspases .
Temporal Effects in Laboratory Settings
In laboratory settings, the effects of this compound change over time. This compound is relatively stable, but its degradation products can also exert toxic effects. Long-term exposure to this compound has been shown to cause persistent oxidative stress, mitochondrial damage, and apoptosis in renal cells . In zebrafish embryos, this compound exposure leads to significant changes in the activities of antioxidant enzymes and the expression of genes related to oxidative stress and apoptosis .
Dosage Effects in Animal Models
The effects of this compound vary with different dosages in animal models. At low doses, this compound can cause bioaccumulation and neurotoxicity, while higher doses result in more severe oxidative stress, tissue damage, and apoptosis . In piglets, this compound exposure led to renal oxidative stress and mitochondrial apoptosis, with vitamin E shown to ameliorate these adverse effects . In zebrafish embryos, this compound exhibited dose-dependent toxicity, with higher doses causing more significant changes in biochemical and molecular indicators .
Metabolic Pathways
This compound is involved in several metabolic pathways, including those related to oxidative stress and apoptosis. It interacts with enzymes such as acetylcholinesterase, superoxide dismutase, and catalase, affecting their activities and leading to the generation of ROS . This compound also influences metabolic flux and metabolite levels, contributing to its toxic effects on cells and tissues .
Transport and Distribution
This compound is transported and distributed within cells and tissues through various mechanisms. It can be absorbed into the blood and circulated to target organs, where it exerts its toxic effects . This compound interacts with transporters and binding proteins, affecting its localization and accumulation in specific tissues . In zebrafish embryos, this compound was shown to accumulate in various tissues, leading to oxidative stress and apoptosis .
Subcellular Localization
This compound’s subcellular localization plays a crucial role in its activity and function. It primarily targets mitochondria, where it induces oxidative stress and apoptosis . This compound’s effects on mitochondrial function are mediated by its interactions with mitochondrial proteins and the generation of ROS . Additionally, this compound’s localization to specific cellular compartments can influence its overall toxicity and impact on cellular processes .
準備方法
Synthetic Routes and Reaction Conditions: Phoxim is typically synthesized using methanol or ethanol as the starting material to generate α-cyanobenzaldehyde oxime sodium. This intermediate is then reacted with diethyl chlorothiophosphate to produce this compound . A green method for the preparation of this compound involves using benzyl alcohol as the starting material, which offers advantages such as higher safety and reduced waste production .
Industrial Production Methods: In industrial settings, this compound is produced by reacting ethyl nitrite with phenylacetonitrile to prepare sodium 2-cyanobenzoxime. This intermediate is then reacted with trichlorothiophosphate and ethanol to synthesize this compound .
化学反応の分析
反応の種類: ホキシムは、酸化、還元、置換などのさまざまな化学反応を起こします。 特に強酸や強塩基の存在下で反応性が高いです .
一般的な試薬と条件: ホキシムを含む反応に使用される一般的な試薬には、ジエチルクロロチオホスフェート、ベンジルアルコール、亜硝酸ナトリウムが含まれます。 反応は通常、安全性を確保し、高収率を得るために、制御された温度と圧力条件下で行われます .
主な生成物: ホキシムを含む反応から生成される主な生成物には、ジエチルリン酸、ホキシムカルボン酸、O,O-ジエチルホスホロチオ酸などがあります .
科学研究における用途
ホキシムは、科学研究において幅広い用途があります。化学では、さまざまな化合物の合成のための試薬として使用されます。生物学では、ホキシムは、有機リン系殺虫剤がさまざまな生物に与える影響を研究するために使用されます。医学では、寄生虫感染症の治療法を開発するために使用されています。 産業では、ホキシムは殺虫剤として使用され、農作物や家畜を害虫から保護しています .
科学的研究の応用
Phoxim has a wide range of applications in scientific research. In chemistry, it is used as a reagent for the synthesis of various compounds. In biology, this compound is used to study the effects of organophosphorus insecticides on different organisms. In medicine, it is used to develop treatments for parasitic infections. In industry, this compound is used as an insecticide to protect crops and livestock from pests .
類似化合物との比較
Phoxim is similar to other organophosphorus insecticides such as chlorpyrifos and pyridaben. this compound is unique in its high efficiency and low toxicity, making it a preferred choice for agricultural and veterinary applications . Other similar compounds include tebufenozide and malathion, which also belong to the organophosphorus class of insecticides .
References
特性
IUPAC Name |
N-diethoxyphosphinothioyloxybenzenecarboximidoyl cyanide | |
---|---|---|
Details | Computed by Lexichem TK 2.7.0 (PubChem release 2021.05.07) | |
Source | PubChem | |
URL | https://pubchem.ncbi.nlm.nih.gov | |
Description | Data deposited in or computed by PubChem | |
InChI |
InChI=1S/C12H15N2O3PS/c1-3-15-18(19,16-4-2)17-14-12(10-13)11-8-6-5-7-9-11/h5-9H,3-4H2,1-2H3 | |
Details | Computed by InChI 1.0.6 (PubChem release 2021.05.07) | |
Source | PubChem | |
URL | https://pubchem.ncbi.nlm.nih.gov | |
Description | Data deposited in or computed by PubChem | |
InChI Key |
ATROHALUCMTWTB-UHFFFAOYSA-N | |
Details | Computed by InChI 1.0.6 (PubChem release 2021.05.07) | |
Source | PubChem | |
URL | https://pubchem.ncbi.nlm.nih.gov | |
Description | Data deposited in or computed by PubChem | |
Canonical SMILES |
CCOP(=S)(OCC)ON=C(C#N)C1=CC=CC=C1 | |
Details | Computed by OEChem 2.3.0 (PubChem release 2021.05.07) | |
Source | PubChem | |
URL | https://pubchem.ncbi.nlm.nih.gov | |
Description | Data deposited in or computed by PubChem | |
Molecular Formula |
C12H15N2O3PS | |
Details | Computed by PubChem 2.1 (PubChem release 2021.05.07) | |
Source | PubChem | |
URL | https://pubchem.ncbi.nlm.nih.gov | |
Description | Data deposited in or computed by PubChem | |
DSSTOX Substance ID |
DTXSID8034324 | |
Record name | Phoxim | |
Source | EPA DSSTox | |
URL | https://comptox.epa.gov/dashboard/DTXSID8034324 | |
Description | DSSTox provides a high quality public chemistry resource for supporting improved predictive toxicology. | |
Molecular Weight |
298.30 g/mol | |
Details | Computed by PubChem 2.1 (PubChem release 2021.05.07) | |
Source | PubChem | |
URL | https://pubchem.ncbi.nlm.nih.gov | |
Description | Data deposited in or computed by PubChem | |
CAS No. |
14816-18-3 | |
Record name | Phoxim | |
Source | CAS Common Chemistry | |
URL | https://commonchemistry.cas.org/detail?cas_rn=14816-18-3 | |
Description | CAS Common Chemistry is an open community resource for accessing chemical information. Nearly 500,000 chemical substances from CAS REGISTRY cover areas of community interest, including common and frequently regulated chemicals, and those relevant to high school and undergraduate chemistry classes. This chemical information, curated by our expert scientists, is provided in alignment with our mission as a division of the American Chemical Society. | |
Explanation | The data from CAS Common Chemistry is provided under a CC-BY-NC 4.0 license, unless otherwise stated. | |
Record name | Phoxim | |
Source | EPA DSSTox | |
URL | https://comptox.epa.gov/dashboard/DTXSID8034324 | |
Description | DSSTox provides a high quality public chemistry resource for supporting improved predictive toxicology. | |
Record name | Phoxim | |
Source | European Chemicals Agency (ECHA) | |
URL | https://echa.europa.eu/substance-information/-/substanceinfo/100.035.337 | |
Description | The European Chemicals Agency (ECHA) is an agency of the European Union which is the driving force among regulatory authorities in implementing the EU's groundbreaking chemicals legislation for the benefit of human health and the environment as well as for innovation and competitiveness. | |
Explanation | Use of the information, documents and data from the ECHA website is subject to the terms and conditions of this Legal Notice, and subject to other binding limitations provided for under applicable law, the information, documents and data made available on the ECHA website may be reproduced, distributed and/or used, totally or in part, for non-commercial purposes provided that ECHA is acknowledged as the source: "Source: European Chemicals Agency, http://echa.europa.eu/". Such acknowledgement must be included in each copy of the material. ECHA permits and encourages organisations and individuals to create links to the ECHA website under the following cumulative conditions: Links can only be made to webpages that provide a link to the Legal Notice page. | |
Record name | PHOXIM | |
Source | FDA Global Substance Registration System (GSRS) | |
URL | https://gsrs.ncats.nih.gov/ginas/app/beta/substances/6F5V775VPO | |
Description | The FDA Global Substance Registration System (GSRS) enables the efficient and accurate exchange of information on what substances are in regulated products. Instead of relying on names, which vary across regulatory domains, countries, and regions, the GSRS knowledge base makes it possible for substances to be defined by standardized, scientific descriptions. | |
Explanation | Unless otherwise noted, the contents of the FDA website (www.fda.gov), both text and graphics, are not copyrighted. They are in the public domain and may be republished, reprinted and otherwise used freely by anyone without the need to obtain permission from FDA. Credit to the U.S. Food and Drug Administration as the source is appreciated but not required. | |
Retrosynthesis Analysis
AI-Powered Synthesis Planning: Our tool employs the Template_relevance Pistachio, Template_relevance Bkms_metabolic, Template_relevance Pistachio_ringbreaker, Template_relevance Reaxys, Template_relevance Reaxys_biocatalysis model, leveraging a vast database of chemical reactions to predict feasible synthetic routes.
One-Step Synthesis Focus: Specifically designed for one-step synthesis, it provides concise and direct routes for your target compounds, streamlining the synthesis process.
Accurate Predictions: Utilizing the extensive PISTACHIO, BKMS_METABOLIC, PISTACHIO_RINGBREAKER, REAXYS, REAXYS_BIOCATALYSIS database, our tool offers high-accuracy predictions, reflecting the latest in chemical research and data.
Strategy Settings
Precursor scoring | Relevance Heuristic |
---|---|
Min. plausibility | 0.01 |
Model | Template_relevance |
Template Set | Pistachio/Bkms_metabolic/Pistachio_ringbreaker/Reaxys/Reaxys_biocatalysis |
Top-N result to add to graph | 6 |
Feasible Synthetic Routes
Q1: What is the primary mechanism of action of Phoxim?
A1: this compound exerts its insecticidal effects by inhibiting acetylcholinesterase (AChE) [, , ]. This enzyme is crucial for breaking down the neurotransmitter acetylcholine (ACh) in the nervous system.
Q2: What are the downstream consequences of this compound-induced AChE inhibition?
A2: Inhibition of AChE leads to an accumulation of acetylcholine (ACh) in the synaptic cleft []. This excess ACh causes continuous stimulation of nerve cells, leading to a range of neurological effects, ultimately culminating in paralysis and death in target insects.
Q3: Does this compound affect other enzymes besides AChE?
A3: Yes, research suggests that this compound exposure can also impact other enzymes, including those involved in oxidative stress responses [, , ]. For example, it has been shown to affect the activity of enzymes like superoxide dismutase (SOD), catalase (CAT), and glutathione S-transferase (GST) [, ].
Q4: What evidence exists for this compound inducing oxidative stress?
A4: Studies have shown that this compound exposure leads to increased levels of malondialdehyde (MDA) in various tissues, including the fat body and midgut of silkworms [, ]. MDA is a well-established marker of lipid peroxidation and oxidative stress.
Q5: What is the molecular formula and weight of this compound?
A5: this compound has the molecular formula C12H15N2O3PS and a molecular weight of 298.3 g/mol.
Q6: Has research explored the use of this compound in controlled-release formulations?
A6: Yes, studies have investigated the incorporation of this compound into organobentonite-based formulations to achieve controlled release []. These formulations aim to reduce the release rate of this compound, potentially minimizing environmental impact and enhancing its efficacy.
Q7: Does the structure of this compound offer insights into its activity?
A7: While specific SAR studies are not detailed in the abstracts, the presence of a thiophosphoryl group is a common feature in organophosphorus insecticides and plays a key role in the interaction with AChE []. Modifications to this and other structural elements can impact this compound's potency and selectivity.
Q8: How does light exposure affect this compound stability?
A8: Research indicates that this compound is photosensitive and degrades under UV irradiation [, ]. This highlights the importance of storage conditions and potential challenges for its application in environments with high UV exposure.
Q9: What are the implications of this compound's environmental persistence?
A9: this compound's persistence in soil and water raises concerns about its potential impact on non-target organisms [, ]. This highlights the need for responsible use, proper waste management, and exploration of alternative pest control methods.
Q10: How is this compound metabolized in target organisms?
A10: Studies have identified several metabolites of this compound, including Phoxom, Phoxom dimer, and various oxidized derivatives [, ]. These metabolites can contribute to the overall toxicity and persistence of this compound in the environment.
Q11: Is there evidence of this compound bioaccumulation?
A11: Yes, research on fish exposed to this compound suggests that it can accumulate in tissues over time, raising concerns about its potential impact on food chains and ecosystems [].
Q12: What model organisms are used to study this compound's effects?
A12: A range of model organisms, including zebrafish, rats, rabbits, silkworms, and mites, are used to assess the toxicity and efficacy of this compound [, , , , , ].
Q13: What types of in vitro studies are conducted with this compound?
A13: Researchers utilize various in vitro models, such as primary-cultured hepatocytes and skin fibroblasts, to investigate the cellular and molecular mechanisms of this compound toxicity [, ]. These studies provide valuable insights into the compound's effects at the cellular level.
Q14: Has resistance to this compound been observed in pest populations?
A14: Yes, resistance to this compound has been documented in several insect species, including the cotton bollworm (Helicoverpa armigera) and the citrus red mite (Panonychus citri) [, , ]. This resistance poses a significant challenge for pest control and underscores the need for resistance management strategies.
Q15: What are the mechanisms underlying this compound resistance?
A15: Research suggests that resistance to this compound can involve various mechanisms, including enhanced detoxification by enzymes like esterases and oxidases [, , ]. Target site insensitivity, where mutations in AChE reduce its binding affinity for this compound, is another potential mechanism of resistance [, ].
Q16: Does this compound resistance confer cross-resistance to other insecticides?
A16: Yes, cross-resistance to other organophosphorus insecticides and even insecticides from different chemical classes, such as pyrethroids, has been reported [, , , ]. This highlights the complex nature of insecticide resistance and the need for integrated pest management approaches.
Q17: What are the toxicological effects of this compound in non-target organisms?
A17: Studies have shown that this compound can induce a range of toxic effects in non-target organisms, including oxidative stress, DNA damage, and apoptosis [, , ]. The severity of these effects varies depending on the dose, duration of exposure, and species.
Q18: How does this compound degrade in the environment?
A18: this compound degradation in the environment is influenced by factors such as pH, temperature, and UV irradiation [, ]. Biodegradation by microorganisms also contributes to its breakdown in soil and water [, ]. Understanding these degradation pathways is crucial for assessing the environmental persistence and potential risks of this compound.
Q19: What analytical methods are used to measure this compound residues?
A19: Various analytical techniques, including gas chromatography (GC), high-performance liquid chromatography (HPLC), and liquid chromatography-tandem mass spectrometry (LC-MS/MS), are employed for the detection and quantification of this compound residues in environmental and biological samples [, , ].
試験管内研究製品の免責事項と情報
BenchChemで提示されるすべての記事および製品情報は、情報提供を目的としています。BenchChemで購入可能な製品は、生体外研究のために特別に設計されています。生体外研究は、ラテン語の "in glass" に由来し、生物体の外で行われる実験を指します。これらの製品は医薬品または薬として分類されておらず、FDAから任何の医療状態、病気、または疾患の予防、治療、または治癒のために承認されていません。これらの製品を人間または動物に体内に導入する形態は、法律により厳格に禁止されています。これらのガイドラインに従うことは、研究と実験において法的および倫理的な基準の遵守を確実にするために重要です。