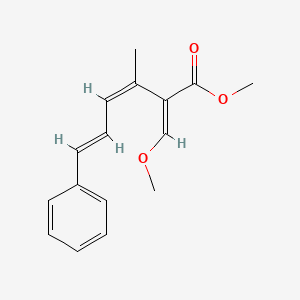
ムシジン
概要
説明
. It belongs to the strobilurin family of bioactive metabolites, which are known for their potent antifungal properties. Mucidin has been commercialized under the name “mucidermin” for the treatment of skin infections .
科学的研究の応用
Mucidin has a wide range of scientific research applications:
Chemistry: Mucidin serves as a lead compound for the development of new antifungal agents.
Biology: Mucidin is used to study fungal metabolism and the mechanisms of antifungal resistance.
Industry: Mucidin is used in agriculture as a fungicide to protect crops from fungal diseases.
作用機序
Target of Action
Mucidin, also known as Strobilurin A, primarily targets the cytochrome bc1 complex in the mitochondrial respiratory chain of fungi . This complex plays a crucial role in cellular respiration, a process vital for energy production in cells.
Mode of Action
Mucidin acts as a potent inhibitor of the cytochrome bc1 complex . It binds to this complex and inhibits electron transfer, disrupting the normal respiration process of fungi . This disruption prevents the fungi from producing the energy they need to grow and reproduce, effectively stopping the spread of fungal infections .
Biochemical Pathways
The inhibition of the cytochrome bc1 complex by Mucidin affects the electron transport chain, a key biochemical pathway in cellular respiration . This disruption leads to a decrease in ATP production, which is essential for various cellular functions. The downstream effects include the inhibition of fungal growth and reproduction .
Result of Action
The primary result of Mucidin’s action is the inhibition of fungal growth and reproduction. By disrupting the normal respiration process of fungi, Mucidin prevents the fungi from producing the energy they need to grow and reproduce . This makes Mucidin an effective antifungal agent.
Action Environment
Environmental factors can influence the action, efficacy, and stability of Mucidin. For instance, the presence of glucose has been shown to affect the action of Mucidin . Additionally, the compound’s action can be influenced by the specific characteristics of the environment in which the fungi live.
生化学分析
Biochemical Properties
Mucidin plays a crucial role in biochemical reactions by inhibiting the electron transport chain in mitochondriaBy binding to the Qo site of the cytochrome bc1 complex, Mucidin disrupts the transfer of electrons from ubiquinol to cytochrome c, leading to the inhibition of ATP synthesis and subsequent cell death . This interaction is highly specific, and Mucidin does not affect other components of the electron transport chain, such as cytochrome b or cytochrome c oxidase .
Cellular Effects
Mucidin exerts significant effects on various types of cells and cellular processes. In yeast cells, Mucidin has been shown to inhibit the growth of Saccharomyces cerevisiae by blocking mitochondrial respiration . This inhibition leads to a decrease in ATP production, which in turn affects various cellular functions, including cell signaling pathways, gene expression, and cellular metabolism . Mucidin-resistant mutants of Saccharomyces cerevisiae have been isolated and characterized, revealing that resistance is often due to mutations in mitochondrial genes encoding components of the cytochrome bc1 complex .
Molecular Mechanism
The molecular mechanism of Mucidin involves its binding to the Qo site of the cytochrome bc1 complex in the mitochondrial inner membrane . This binding prevents the transfer of electrons from ubiquinol to cytochrome c, effectively halting the electron transport chain and ATP synthesis . The inhibition of ATP synthesis leads to a cascade of cellular events, including the activation of stress response pathways, changes in gene expression, and ultimately cell death . Mucidin’s specificity for the Qo site of the cytochrome bc1 complex is due to its unique chemical structure, which allows it to fit precisely into the binding pocket .
Temporal Effects in Laboratory Settings
In laboratory settings, the effects of Mucidin have been observed to change over time. Studies have shown that Mucidin is relatively stable under various conditions, but its efficacy can decrease over prolonged exposure due to degradation or the development of resistance . Long-term exposure to Mucidin in in vitro and in vivo studies has revealed that it can lead to adaptive changes in cellular function, including alterations in mitochondrial structure and function . These changes can affect the overall metabolic state of the cell and its ability to respond to environmental stressors .
Dosage Effects in Animal Models
The effects of Mucidin vary with different dosages in animal models. At low doses, Mucidin has been shown to effectively inhibit fungal growth without causing significant toxicity . At higher doses, Mucidin can exhibit toxic effects, including damage to mitochondrial function and induction of oxidative stress . Studies in animal models have identified threshold doses at which Mucidin transitions from being an effective antifungal agent to a toxic compound . These findings highlight the importance of carefully controlling the dosage of Mucidin in therapeutic applications .
Metabolic Pathways
Mucidin is involved in several metabolic pathways, primarily related to its role in inhibiting mitochondrial respiration . By targeting the cytochrome bc1 complex, Mucidin disrupts the normal flow of electrons through the electron transport chain, leading to a decrease in ATP production and an increase in reactive oxygen species (ROS) production . This disruption can affect various metabolic processes, including glycolysis, the tricarboxylic acid (TCA) cycle, and oxidative phosphorylation . Additionally, Mucidin’s interaction with the cytochrome bc1 complex can influence the levels of key metabolites, such as NADH and FADH2, which are essential for cellular energy production .
Transport and Distribution
Within cells and tissues, Mucidin is transported and distributed through various mechanisms. It can diffuse across cell membranes due to its lipophilic nature, allowing it to reach its target site in the mitochondrial inner membrane . Mucidin may also interact with specific transporters or binding proteins that facilitate its uptake and distribution within the cell . Once inside the cell, Mucidin accumulates in the mitochondria, where it exerts its inhibitory effects on the cytochrome bc1 complex . The distribution of Mucidin within tissues can vary depending on factors such as tissue type, blood flow, and the presence of transporters .
Subcellular Localization
Mucidin’s subcellular localization is primarily within the mitochondria, where it targets the cytochrome bc1 complex . The compound’s lipophilic nature allows it to readily cross the mitochondrial membranes and accumulate in the inner membrane, where it binds to the Qo site of the cytochrome bc1 complex . This specific localization is crucial for Mucidin’s inhibitory effects on mitochondrial respiration and ATP synthesis . Additionally, Mucidin’s activity may be influenced by post-translational modifications or interactions with other mitochondrial proteins that affect its binding affinity and efficacy .
準備方法
Synthetic Routes and Reaction Conditions: Mucidin can be synthesized through a series of organic reactions involving the formation of a triene system. The synthetic route typically involves the use of a benzoate starter unit derived from phenylalanine via cinnamate . The process includes the formation of a linear tetraketide, followed by a key rearrangement involving an epoxide intermediate to form the β-methoxyacrylate moiety essential for its biological activity .
Industrial Production Methods: Industrial production of mucidin involves fermentation processes using the basidiomycete Oudemansiella mucida. The fermentation broth is then subjected to extraction and purification processes to isolate mucidin in its crystalline form .
化学反応の分析
Types of Reactions: Mucidin undergoes various chemical reactions, including:
Oxidation: Mucidin can be oxidized to form different derivatives with altered biological activities.
Reduction: Reduction reactions can modify the double bonds in the triene system.
Common Reagents and Conditions:
Oxidation: Common oxidizing agents include potassium permanganate and hydrogen peroxide.
Reduction: Reducing agents such as sodium borohydride and lithium aluminum hydride are used.
Substitution: Reagents like halogens and alkylating agents are employed under controlled conditions.
Major Products: The major products formed from these reactions include various strobilurin analogues with modified antifungal activities .
類似化合物との比較
- Nystatin
- Pimaricin
- Myxothiazol
- Fluconazole
- Itaconic acid
- Quercetin
- Cinnamic acid
- Rutin
- Caffeic acid
- Gallic acid
- Apigenin
- Rosmarinic acid
Mucidin continues to be a valuable compound in scientific research and industrial applications due to its potent antifungal properties and unique mechanism of action.
特性
IUPAC Name |
methyl (2E,3Z,5E)-2-(methoxymethylidene)-3-methyl-6-phenylhexa-3,5-dienoate | |
---|---|---|
Source | PubChem | |
URL | https://pubchem.ncbi.nlm.nih.gov | |
Description | Data deposited in or computed by PubChem | |
InChI |
InChI=1S/C16H18O3/c1-13(15(12-18-2)16(17)19-3)8-7-11-14-9-5-4-6-10-14/h4-12H,1-3H3/b11-7+,13-8-,15-12+ | |
Source | PubChem | |
URL | https://pubchem.ncbi.nlm.nih.gov | |
Description | Data deposited in or computed by PubChem | |
InChI Key |
JSCQSBGXKRTPHZ-SYKZHUKTSA-N | |
Source | PubChem | |
URL | https://pubchem.ncbi.nlm.nih.gov | |
Description | Data deposited in or computed by PubChem | |
Canonical SMILES |
CC(=CC=CC1=CC=CC=C1)C(=COC)C(=O)OC | |
Source | PubChem | |
URL | https://pubchem.ncbi.nlm.nih.gov | |
Description | Data deposited in or computed by PubChem | |
Isomeric SMILES |
C/C(=C/C=C/C1=CC=CC=C1)/C(=C\OC)/C(=O)OC | |
Source | PubChem | |
URL | https://pubchem.ncbi.nlm.nih.gov | |
Description | Data deposited in or computed by PubChem | |
Molecular Formula |
C16H18O3 | |
Source | PubChem | |
URL | https://pubchem.ncbi.nlm.nih.gov | |
Description | Data deposited in or computed by PubChem | |
DSSTOX Substance ID |
DTXSID401025570 | |
Record name | Mucidin | |
Source | EPA DSSTox | |
URL | https://comptox.epa.gov/dashboard/DTXSID401025570 | |
Description | DSSTox provides a high quality public chemistry resource for supporting improved predictive toxicology. | |
Molecular Weight |
258.31 g/mol | |
Source | PubChem | |
URL | https://pubchem.ncbi.nlm.nih.gov | |
Description | Data deposited in or computed by PubChem | |
CAS No. |
52110-55-1 | |
Record name | Strobilurin A | |
Source | CAS Common Chemistry | |
URL | https://commonchemistry.cas.org/detail?cas_rn=52110-55-1 | |
Description | CAS Common Chemistry is an open community resource for accessing chemical information. Nearly 500,000 chemical substances from CAS REGISTRY cover areas of community interest, including common and frequently regulated chemicals, and those relevant to high school and undergraduate chemistry classes. This chemical information, curated by our expert scientists, is provided in alignment with our mission as a division of the American Chemical Society. | |
Explanation | The data from CAS Common Chemistry is provided under a CC-BY-NC 4.0 license, unless otherwise stated. | |
Record name | Mucidin | |
Source | ChemIDplus | |
URL | https://pubchem.ncbi.nlm.nih.gov/substance/?source=chemidplus&sourceid=0052110551 | |
Description | ChemIDplus is a free, web search system that provides access to the structure and nomenclature authority files used for the identification of chemical substances cited in National Library of Medicine (NLM) databases, including the TOXNET system. | |
Record name | Mucidin | |
Source | EPA DSSTox | |
URL | https://comptox.epa.gov/dashboard/DTXSID401025570 | |
Description | DSSTox provides a high quality public chemistry resource for supporting improved predictive toxicology. | |
Record name | MUCIDIN | |
Source | FDA Global Substance Registration System (GSRS) | |
URL | https://gsrs.ncats.nih.gov/ginas/app/beta/substances/15143I0275 | |
Description | The FDA Global Substance Registration System (GSRS) enables the efficient and accurate exchange of information on what substances are in regulated products. Instead of relying on names, which vary across regulatory domains, countries, and regions, the GSRS knowledge base makes it possible for substances to be defined by standardized, scientific descriptions. | |
Explanation | Unless otherwise noted, the contents of the FDA website (www.fda.gov), both text and graphics, are not copyrighted. They are in the public domain and may be republished, reprinted and otherwise used freely by anyone without the need to obtain permission from FDA. Credit to the U.S. Food and Drug Administration as the source is appreciated but not required. | |
Retrosynthesis Analysis
AI-Powered Synthesis Planning: Our tool employs the Template_relevance Pistachio, Template_relevance Bkms_metabolic, Template_relevance Pistachio_ringbreaker, Template_relevance Reaxys, Template_relevance Reaxys_biocatalysis model, leveraging a vast database of chemical reactions to predict feasible synthetic routes.
One-Step Synthesis Focus: Specifically designed for one-step synthesis, it provides concise and direct routes for your target compounds, streamlining the synthesis process.
Accurate Predictions: Utilizing the extensive PISTACHIO, BKMS_METABOLIC, PISTACHIO_RINGBREAKER, REAXYS, REAXYS_BIOCATALYSIS database, our tool offers high-accuracy predictions, reflecting the latest in chemical research and data.
Strategy Settings
Precursor scoring | Relevance Heuristic |
---|---|
Min. plausibility | 0.01 |
Model | Template_relevance |
Template Set | Pistachio/Bkms_metabolic/Pistachio_ringbreaker/Reaxys/Reaxys_biocatalysis |
Top-N result to add to graph | 6 |
Feasible Synthetic Routes
試験管内研究製品の免責事項と情報
BenchChemで提示されるすべての記事および製品情報は、情報提供を目的としています。BenchChemで購入可能な製品は、生体外研究のために特別に設計されています。生体外研究は、ラテン語の "in glass" に由来し、生物体の外で行われる実験を指します。これらの製品は医薬品または薬として分類されておらず、FDAから任何の医療状態、病気、または疾患の予防、治療、または治癒のために承認されていません。これらの製品を人間または動物に体内に導入する形態は、法律により厳格に禁止されています。これらのガイドラインに従うことは、研究と実験において法的および倫理的な基準の遵守を確実にするために重要です。