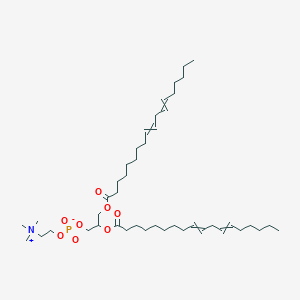
1,2-ジリノレオイル-SN-グリセロ-3-ホスホコリン
概要
説明
1,2-Dilinoleoyl-SN-glycero-3-phosphocholine is a phospholipid compound with the molecular formula C44H80NO8P and a molecular weight of 782.08 g/mol . It is a derivative of phosphatidylcholine, where two linoleic acid molecules are esterified to the glycerol backbone. This compound is commonly used in the study of biological membranes due to its structural similarity to natural phospholipids .
科学的研究の応用
1,2-Dilinoleoyl-SN-glycero-3-phosphocholine is extensively used in scientific research due to its structural and functional properties:
Biological Membrane Studies: It is used to simulate and study the properties of biological membranes, particularly how varying degrees of lipid unsaturation influence membrane stability, permeability, and the formation of lipid domains or rafts.
Obesity Research: It has been shown to increase insulin sensitivity in palmitate-treated myotubes and induce lipolysis in adipocytes, making it a valuable compound in obesity research.
Cryopreservation: It is used in the formulation of liposomes for the cryopreservation of red blood cells.
作用機序
Target of Action
The primary targets of 1,2-Dilinoleoyl-SN-glycero-3-phosphocholine (DLPC) are adipocytes and muscle cells . In adipocytes, DLPC induces lipolysis, the breakdown of fats and other lipids to release fatty acids . In muscle cells, DLPC increases insulin sensitivity .
Mode of Action
DLPC interacts with its targets by enhancing the release of tumor necrosis factor α (TNF-α) in adipocytes . This cytokine plays a crucial role in cell signaling and can induce lipolysis and apoptosis . In muscle cells, DLPC increases the expression levels of peroxisome proliferator-activated receptor α (PPARα) , a key regulator of lipid metabolism and inflammation .
Biochemical Pathways
DLPC affects several biochemical pathways. In adipocytes, it enhances lipolysis and apoptosis via a TNFα-dependent pathway . In muscle cells, DLPC attenuates palmitate-induced inflammation and insulin resistance through a PPARα-mediated pathway .
Pharmacokinetics
Phospholipids like dlpc are known to form stable bilayers and vesicles, which can encapsulate drugs and deliver them to specific targets in the body . As a stabilizer and emulsifier, DLPC can enhance the solubility and bioavailability of drugs .
Result of Action
The action of DLPC results in enhanced lipolysis and apoptosis in adipocytes, leading to the breakdown of fats . In muscle cells, DLPC attenuates inflammation and insulin resistance, thereby increasing insulin sensitivity . These actions make DLPC a potentially effective substance for the treatment of obesity-mediated disorders such as impaired fat metabolism and insulin resistance .
Action Environment
Like other phospholipids, dlpc’s ability to form stable bilayers and vesicles may be influenced by factors such as temperature, ph, and the presence of other substances .
生化学分析
Biochemical Properties
1,2-Dilinoleoyl-SN-glycero-3-phosphocholine is known for its role in enhancing insulin sensitivity and inducing lipolysis in adipocytes. It interacts with several enzymes and proteins, including peroxisome proliferator-activated receptor alpha (PPARα) and tumor necrosis factor alpha (TNF-α). The interaction with PPARα helps in the suppression of inflammation and insulin resistance in muscle cells, while the interaction with TNF-α promotes lipolysis and apoptosis in adipocytes .
Cellular Effects
1,2-Dilinoleoyl-SN-glycero-3-phosphocholine has been shown to influence various cellular processes. In adipocytes, it enhances lipolysis and apoptosis through a TNF-α-dependent pathway. In muscle cells, it increases insulin sensitivity by suppressing inflammation through PPARα-mediated pathways. Additionally, it has been reported to induce browning of white adipose tissue, which is beneficial for energy homeostasis .
Molecular Mechanism
At the molecular level, 1,2-Dilinoleoyl-SN-glycero-3-phosphocholine exerts its effects by binding to specific receptors and modulating signaling pathways. It activates PPARα, which leads to the suppression of inflammation and improvement of insulin sensitivity. It also promotes the release of TNF-α in adipocytes, which in turn induces lipolysis and apoptosis. These interactions highlight the compound’s role in regulating metabolic processes and maintaining cellular homeostasis .
Temporal Effects in Laboratory Settings
In laboratory settings, the effects of 1,2-Dilinoleoyl-SN-glycero-3-phosphocholine have been observed to change over time. The compound is relatively stable, but its long-term effects on cellular function have been studied in both in vitro and in vivo settings. For instance, prolonged exposure to 1,2-Dilinoleoyl-SN-glycero-3-phosphocholine has been shown to maintain its lipolytic and insulin-sensitizing effects, indicating its potential for long-term therapeutic applications .
Dosage Effects in Animal Models
The effects of 1,2-Dilinoleoyl-SN-glycero-3-phosphocholine vary with different dosages in animal models. At lower doses, it effectively enhances insulin sensitivity and induces lipolysis without causing adverse effects. At higher doses, there may be potential toxic effects, including excessive lipolysis and apoptosis in adipocytes. These findings suggest the importance of optimizing dosage to achieve therapeutic benefits while minimizing adverse effects .
Metabolic Pathways
1,2-Dilinoleoyl-SN-glycero-3-phosphocholine is involved in several metabolic pathways. It interacts with enzymes such as PPARα and TNF-α, which play crucial roles in lipid metabolism and inflammation. The compound’s involvement in these pathways helps regulate metabolic flux and maintain energy homeostasis. Additionally, it has been shown to influence the levels of various metabolites, further highlighting its role in metabolic regulation .
Transport and Distribution
Within cells and tissues, 1,2-Dilinoleoyl-SN-glycero-3-phosphocholine is transported and distributed through specific transporters and binding proteins. These interactions facilitate its localization and accumulation in target tissues, such as adipose tissue and muscle cells. The compound’s distribution is crucial for its biological activity and therapeutic potential .
Subcellular Localization
1,2-Dilinoleoyl-SN-glycero-3-phosphocholine is primarily localized in the cell membrane, where it contributes to membrane structure and function. It may also be found in other subcellular compartments, depending on its interactions with specific targeting signals and post-translational modifications. These localizations are essential for its activity and function in various cellular processes .
準備方法
Synthetic Routes and Reaction Conditions
1,2-Dilinoleoyl-SN-glycero-3-phosphocholine can be synthesized through a series of esterification and phosphorylation reactions starting from linoleic acid . The general synthetic route involves:
Esterification: Linoleic acid is esterified with glycerol to form 1,2-dilinoleoyl-glycerol.
Phosphorylation: The 1,2-dilinoleoyl-glycerol is then phosphorylated using phosphocholine chloride in the presence of a base such as triethylamine.
Industrial Production Methods
Industrial production of 1,2-Dilinoleoyl-SN-glycero-3-phosphocholine typically involves large-scale esterification and phosphorylation processes. The reaction conditions are optimized to ensure high yield and purity, often involving the use of catalysts and controlled temperature and pressure conditions .
化学反応の分析
Types of Reactions
1,2-Dilinoleoyl-SN-glycero-3-phosphocholine undergoes various chemical reactions, including:
Common Reagents and Conditions
Major Products Formed
Oxidation: Hydroperoxides, aldehydes, and ketones.
Hydrolysis: Linoleic acid and glycerophosphocholine.
類似化合物との比較
Similar Compounds
1,2-Dioleoyl-SN-glycero-3-phosphocholine: Similar in structure but contains oleic acid instead of linoleic acid.
1,2-Dioctanoyl-SN-glycero-3-phosphocholine: Contains octanoic acid chains, making it more hydrophobic.
Uniqueness
1,2-Dilinoleoyl-SN-glycero-3-phosphocholine is unique due to its high degree of unsaturation, which significantly influences membrane properties and biological activities. Its ability to induce white adipose browning and increase insulin sensitivity sets it apart from other phospholipids .
特性
IUPAC Name |
[(2R)-2,3-bis[[(9Z,12Z)-octadeca-9,12-dienoyl]oxy]propyl] 2-(trimethylazaniumyl)ethyl phosphate | |
---|---|---|
Source | PubChem | |
URL | https://pubchem.ncbi.nlm.nih.gov | |
Description | Data deposited in or computed by PubChem | |
InChI |
InChI=1S/C44H80NO8P/c1-6-8-10-12-14-16-18-20-22-24-26-28-30-32-34-36-43(46)50-40-42(41-52-54(48,49)51-39-38-45(3,4)5)53-44(47)37-35-33-31-29-27-25-23-21-19-17-15-13-11-9-7-2/h14-17,20-23,42H,6-13,18-19,24-41H2,1-5H3/b16-14-,17-15-,22-20-,23-21-/t42-/m1/s1 | |
Source | PubChem | |
URL | https://pubchem.ncbi.nlm.nih.gov | |
Description | Data deposited in or computed by PubChem | |
InChI Key |
FVXDQWZBHIXIEJ-LNDKUQBDSA-N | |
Source | PubChem | |
URL | https://pubchem.ncbi.nlm.nih.gov | |
Description | Data deposited in or computed by PubChem | |
Canonical SMILES |
CCCCCC=CCC=CCCCCCCCC(=O)OCC(COP(=O)([O-])OCC[N+](C)(C)C)OC(=O)CCCCCCCC=CCC=CCCCCC | |
Source | PubChem | |
URL | https://pubchem.ncbi.nlm.nih.gov | |
Description | Data deposited in or computed by PubChem | |
Isomeric SMILES |
CCCCC/C=C\C/C=C\CCCCCCCC(=O)OC[C@H](COP(=O)([O-])OCC[N+](C)(C)C)OC(=O)CCCCCCC/C=C\C/C=C\CCCCC | |
Source | PubChem | |
URL | https://pubchem.ncbi.nlm.nih.gov | |
Description | Data deposited in or computed by PubChem | |
Molecular Formula |
C44H80NO8P | |
Source | PubChem | |
URL | https://pubchem.ncbi.nlm.nih.gov | |
Description | Data deposited in or computed by PubChem | |
DSSTOX Substance ID |
DTXSID201260902 | |
Record name | 1,2-Dilinoleoyl-sn-glycero-3-phosphocholine | |
Source | EPA DSSTox | |
URL | https://comptox.epa.gov/dashboard/DTXSID201260902 | |
Description | DSSTox provides a high quality public chemistry resource for supporting improved predictive toxicology. | |
Molecular Weight |
782.1 g/mol | |
Source | PubChem | |
URL | https://pubchem.ncbi.nlm.nih.gov | |
Description | Data deposited in or computed by PubChem | |
Physical Description |
Solid | |
Record name | PC(18:2(9Z,12Z)/18:2(9Z,12Z)) | |
Source | Human Metabolome Database (HMDB) | |
URL | http://www.hmdb.ca/metabolites/HMDB0008138 | |
Description | The Human Metabolome Database (HMDB) is a freely available electronic database containing detailed information about small molecule metabolites found in the human body. | |
Explanation | HMDB is offered to the public as a freely available resource. Use and re-distribution of the data, in whole or in part, for commercial purposes requires explicit permission of the authors and explicit acknowledgment of the source material (HMDB) and the original publication (see the HMDB citing page). We ask that users who download significant portions of the database cite the HMDB paper in any resulting publications. | |
CAS No. |
998-06-1 | |
Record name | 1,2-Dilinoleoyl-sn-glycero-3-phosphocholine | |
Source | CAS Common Chemistry | |
URL | https://commonchemistry.cas.org/detail?cas_rn=998-06-1 | |
Description | CAS Common Chemistry is an open community resource for accessing chemical information. Nearly 500,000 chemical substances from CAS REGISTRY cover areas of community interest, including common and frequently regulated chemicals, and those relevant to high school and undergraduate chemistry classes. This chemical information, curated by our expert scientists, is provided in alignment with our mission as a division of the American Chemical Society. | |
Explanation | The data from CAS Common Chemistry is provided under a CC-BY-NC 4.0 license, unless otherwise stated. | |
Record name | L-Dilinoleoyllecithin | |
Source | ChemIDplus | |
URL | https://pubchem.ncbi.nlm.nih.gov/substance/?source=chemidplus&sourceid=0000998061 | |
Description | ChemIDplus is a free, web search system that provides access to the structure and nomenclature authority files used for the identification of chemical substances cited in National Library of Medicine (NLM) databases, including the TOXNET system. | |
Record name | L-Dilinoleoyllecithin | |
Source | DrugBank | |
URL | https://www.drugbank.ca/drugs/DB04372 | |
Description | The DrugBank database is a unique bioinformatics and cheminformatics resource that combines detailed drug (i.e. chemical, pharmacological and pharmaceutical) data with comprehensive drug target (i.e. sequence, structure, and pathway) information. | |
Explanation | Creative Common's Attribution-NonCommercial 4.0 International License (http://creativecommons.org/licenses/by-nc/4.0/legalcode) | |
Record name | 1,2-Dilinoleoyl-sn-glycero-3-phosphocholine | |
Source | EPA DSSTox | |
URL | https://comptox.epa.gov/dashboard/DTXSID201260902 | |
Description | DSSTox provides a high quality public chemistry resource for supporting improved predictive toxicology. | |
Record name | [R-(all-Z)]-[4-oxido-10-oxo-7-[(1-oxooctadeca-9,12-dienyl)oxy]-3,5,9-trioxa-4-phosphaheptacosa-18,21-dienyl]trimethylammonium 4-oxide | |
Source | European Chemicals Agency (ECHA) | |
URL | https://echa.europa.eu/substance-information/-/substanceinfo/100.012.406 | |
Description | The European Chemicals Agency (ECHA) is an agency of the European Union which is the driving force among regulatory authorities in implementing the EU's groundbreaking chemicals legislation for the benefit of human health and the environment as well as for innovation and competitiveness. | |
Explanation | Use of the information, documents and data from the ECHA website is subject to the terms and conditions of this Legal Notice, and subject to other binding limitations provided for under applicable law, the information, documents and data made available on the ECHA website may be reproduced, distributed and/or used, totally or in part, for non-commercial purposes provided that ECHA is acknowledged as the source: "Source: European Chemicals Agency, http://echa.europa.eu/". Such acknowledgement must be included in each copy of the material. ECHA permits and encourages organisations and individuals to create links to the ECHA website under the following cumulative conditions: Links can only be made to webpages that provide a link to the Legal Notice page. | |
Record name | 1,2-DILINOLEOYL-SN-GLYCERO-3-PHOSPHOCHOLINE | |
Source | FDA Global Substance Registration System (GSRS) | |
URL | https://gsrs.ncats.nih.gov/ginas/app/beta/substances/7L874P1M1O | |
Description | The FDA Global Substance Registration System (GSRS) enables the efficient and accurate exchange of information on what substances are in regulated products. Instead of relying on names, which vary across regulatory domains, countries, and regions, the GSRS knowledge base makes it possible for substances to be defined by standardized, scientific descriptions. | |
Explanation | Unless otherwise noted, the contents of the FDA website (www.fda.gov), both text and graphics, are not copyrighted. They are in the public domain and may be republished, reprinted and otherwise used freely by anyone without the need to obtain permission from FDA. Credit to the U.S. Food and Drug Administration as the source is appreciated but not required. | |
Record name | PC(18:2(9Z,12Z)/18:2(9Z,12Z)) | |
Source | Human Metabolome Database (HMDB) | |
URL | http://www.hmdb.ca/metabolites/HMDB0008138 | |
Description | The Human Metabolome Database (HMDB) is a freely available electronic database containing detailed information about small molecule metabolites found in the human body. | |
Explanation | HMDB is offered to the public as a freely available resource. Use and re-distribution of the data, in whole or in part, for commercial purposes requires explicit permission of the authors and explicit acknowledgment of the source material (HMDB) and the original publication (see the HMDB citing page). We ask that users who download significant portions of the database cite the HMDB paper in any resulting publications. | |
Retrosynthesis Analysis
AI-Powered Synthesis Planning: Our tool employs the Template_relevance Pistachio, Template_relevance Bkms_metabolic, Template_relevance Pistachio_ringbreaker, Template_relevance Reaxys, Template_relevance Reaxys_biocatalysis model, leveraging a vast database of chemical reactions to predict feasible synthetic routes.
One-Step Synthesis Focus: Specifically designed for one-step synthesis, it provides concise and direct routes for your target compounds, streamlining the synthesis process.
Accurate Predictions: Utilizing the extensive PISTACHIO, BKMS_METABOLIC, PISTACHIO_RINGBREAKER, REAXYS, REAXYS_BIOCATALYSIS database, our tool offers high-accuracy predictions, reflecting the latest in chemical research and data.
Strategy Settings
Precursor scoring | Relevance Heuristic |
---|---|
Min. plausibility | 0.01 |
Model | Template_relevance |
Template Set | Pistachio/Bkms_metabolic/Pistachio_ringbreaker/Reaxys/Reaxys_biocatalysis |
Top-N result to add to graph | 6 |
Feasible Synthetic Routes
Q1: What is 1,2-Dilinoleoyl-SN-glycero-3-phosphocholine (1,2-Dilinoleoyl-sn-glycero-3-phosphocholine) and what are its key biological functions?
A1: 1,2-Dilinoleoyl-SN-glycero-3-phosphocholine (1,2-Dilinoleoyl-sn-glycero-3-phosphocholine), often abbreviated as DLPC, is a phospholipid commonly found in biological membranes, particularly in plants like soybeans. It's characterized by two linoleic acid chains attached to the glycerol backbone. Research suggests that DLPC possesses various biological activities, including:
- Anti-inflammatory effects: DLPC has been shown to induce the expression of heme oxygenase-1 (HO-1), an enzyme with anti-inflammatory properties, in macrophages []. This effect is mediated through the activation of the Nrf2 pathway, which regulates cellular defense mechanisms against oxidative stress.
- Improving insulin sensitivity: Studies have demonstrated that DLPC can enhance insulin sensitivity in muscle cells treated with palmitate, a saturated fatty acid known to induce insulin resistance []. This suggests a potential role for DLPC in managing metabolic disorders like type 2 diabetes.
- Promoting adipose browning: Emerging evidence suggests that DLPC secreted from skeletal muscle may contribute to systemic energy homeostasis by promoting the browning of white adipose tissue []. This process involves the conversion of energy-storing white fat cells into energy-burning beige fat cells, potentially impacting obesity and related metabolic conditions.
Q2: How does DLPC interact with biological membranes?
A2: As a phospholipid, DLPC readily incorporates into lipid bilayers, which are the fundamental building blocks of cell membranes. Its two unsaturated linoleic acid chains influence the fluidity and packing of the membrane. This interaction is crucial for various cellular processes, including membrane protein function, signal transduction, and vesicle formation. []
Q3: How does the presence of DLPC in liposomes affect their stability during storage?
A3: Liposomes containing DLPC, due to its unsaturated linoleic acid chains, are susceptible to chemical degradation during long-term storage, particularly at higher temperatures []. This degradation is primarily driven by lipid peroxidation, a process accelerated by factors like temperature, oxygen exposure, and the presence of metal ion contaminants.
Q4: What strategies can be employed to improve the stability of DLPC-containing liposomes during storage?
A4: Several approaches can be used to enhance the stability of liposomes formulated with DLPC:
- Chelation of metal ions: Removing or chelating metal ion contaminants, especially iron, from the formulation can significantly reduce lipid peroxidation and improve stability [].
- Storage temperature control: Storing lyophilized DLPC-containing formulations at lower temperatures (e.g., 4°C) helps slow down degradation rates [].
- Oxygen depletion: Reducing the oxygen content in the sample prior to lyophilization can minimize oxidative degradation [].
Q5: Can DLPC be used as an elicitor to induce plant defense mechanisms against insects?
A5: While DLPC itself hasn't been directly identified as an insect elicitor, studies have shown that certain phospholipids, particularly those with specific fatty acid compositions, can trigger defense responses in plants []. In the case of the white-backed planthopper (Sogatella furcifera), four phospholipids, including 1,2-dilinoleoyl-sn-glycero-3-phosphocholine (DLPC), were isolated and identified as elicitors in the insect that induce the production of an ovicidal substance, benzyl benzoate, in specific Japonica rice plant varieties [].
Q6: How does the structure of DLPC relate to its biological activity?
A6: The presence of two linoleic acid chains in DLPC is crucial for its biological activity. These unsaturated fatty acids influence the fluidity and packing of cell membranes, potentially affecting the function of membrane proteins and signaling pathways. Further research is needed to fully elucidate the structure-activity relationships of DLPC and its derivatives.
Q7: What analytical techniques are commonly used to characterize and quantify DLPC?
A7: Several analytical methods are employed for DLPC analysis:
- High-Performance Liquid Chromatography (HPLC): HPLC, often coupled with an evaporative light scattering detector (ELSD), enables the separation and quantification of individual phosphatidylcholine species, including DLPC []. This technique allows for the determination of DLPC content in various samples.
- Mass Spectrometry (MS): MS techniques like Matrix-Assisted Laser Desorption/Ionization Time-of-Flight (MALDI-TOF) are used to identify and confirm the molecular weight and structure of DLPC [].
- Nuclear Magnetic Resonance (NMR) Spectroscopy: NMR provides detailed structural information about DLPC, aiding in the identification and characterization of its molecular features [].
- Fourier Transform Infrared (FTIR) Spectroscopy: FTIR can be used to study the interactions of DLPC within lipid bilayers, providing insights into its effects on membrane structure and dynamics [, ].
- Raman Spectroscopy: This technique offers insights into the hydrocarbon chain disorder within phospholipid bilayers, including those containing DLPC, by analyzing specific vibrational modes [].
Q8: What are the potential therapeutic applications of DLPC?
A8: The diverse biological activities of DLPC make it a promising candidate for therapeutic development in areas such as:
- Inflammatory diseases: The anti-inflammatory properties of DLPC, mediated by the induction of HO-1, suggest its potential in managing conditions like inflammatory bowel disease and arthritis [].
- Metabolic disorders: DLPC's ability to improve insulin sensitivity in muscle cells and potentially enhance adipose browning highlights its therapeutic potential for treating type 2 diabetes and obesity [, ].
- Neurodegenerative diseases: Research on related phospholipids suggests possible applications in addressing neurodegenerative diseases like Alzheimer's disease [].
Q9: What are the limitations of using DLPC in therapeutic applications?
A9: Despite its therapeutic promise, there are challenges associated with using DLPC:
- Stability: The susceptibility of DLPC to oxidation necessitates the development of stable formulations and appropriate storage conditions to ensure its efficacy. []
Q10: How can computational chemistry and modeling be utilized in DLPC research?
A10: Computational methods are valuable tools for understanding DLPC's behavior and interactions:
- Molecular dynamics (MD) simulations: MD simulations can provide insights into how DLPC incorporates into lipid bilayers, influencing membrane fluidity, structure, and interactions with other molecules [, ].
- Free energy calculations: These calculations can help determine the energetic favorability of DLPC interactions with other molecules, such as drugs or membrane proteins [].
試験管内研究製品の免責事項と情報
BenchChemで提示されるすべての記事および製品情報は、情報提供を目的としています。BenchChemで購入可能な製品は、生体外研究のために特別に設計されています。生体外研究は、ラテン語の "in glass" に由来し、生物体の外で行われる実験を指します。これらの製品は医薬品または薬として分類されておらず、FDAから任何の医療状態、病気、または疾患の予防、治療、または治癒のために承認されていません。これらの製品を人間または動物に体内に導入する形態は、法律により厳格に禁止されています。これらのガイドラインに従うことは、研究と実験において法的および倫理的な基準の遵守を確実にするために重要です。