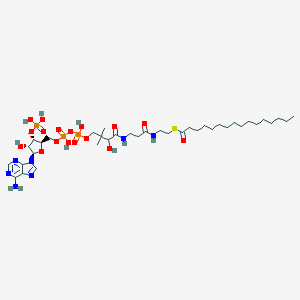
パルミトイルCoA
概要
説明
パルミトイルCoAは、脂質代謝において重要な役割を果たすアシルCoAチオエステルです。これはパルミチン酸の「活性化」型であり、カルニチンシャトルシステムによってミトコンドリアマトリックスに輸送することができます。ミトコンドリア内部では、β酸化に関与します。さらに、this compoundは、スフィンゴ脂質やリン脂質の構成成分であるスフィンゴシンの生合成における基質としても用いられます .
科学的研究の応用
パルミトイルCoAは、科学研究において数多くの応用があります:
化学: 脂質代謝と脂肪酸を含む酵素反応の研究に使用されます。
生物学: タンパク質パルミトイル化を通じて細胞シグナル伝達と膜ダイナミクスにおける役割について調査されています.
作用機序
パルミトイルCoAは、主に脂質代謝における役割を通じてその効果を発揮します。これはβ酸化経路に関与し、そこでアセチルCoAを生成するために分解され、その後クエン酸回路に入りエネルギーを生成します。さらに、this compoundはタンパク質パルミトイル化の基質であり、多くの細胞タンパク質の機能を調節する可逆的な脂質修飾です .
類似化合物:
ステアロイルCoA: ステアリン酸から誘導された別の長鎖アシルCoAチオエステルです。
ミリストイルCoA: ミリスチン酸から誘導され、同様の代謝経路に関与しています。
パルミトレオイルCoA: パルミトレイン酸から誘導され、脂質代謝に関与しています.
独自性: this compoundは、β酸化によるエネルギー生成と様々な細胞プロセスを調節するタンパク質パルミトイル化への関与という、幅広い役割を持つことからユニークです。その二重の機能は、脂質代謝と細胞シグナル伝達における重要な分子としての役割を果たしています .
Safety and Hazards
将来の方向性
Recent research has highlighted the transformative potential of studying palmitoylation in cancer treatment . Palmitoyl-CoA serves an important dual purpose as it is not only a key metabolite fueling energy metabolism, but is also a substrate for this post-translational modification . Therefore, understanding the role of palmitoylation in regulating substrate metabolism could lead to groundbreaking therapeutic strategies .
生化学分析
Biochemical Properties
Palmitoyl-CoA is involved in several biochemical reactions. It is a substrate in the biosynthesis of sphingosine , a type of sphingolipid that plays a key role in signal transmission and cell recognition. Palmitoyl-CoA also participates in beta-oxidation, a metabolic process that breaks down fatty acids to produce energy . The attachment of palmitoyl groups to proteins alters their membrane affinity, changing their subcellular localization, stability, and protein-protein interactions .
Cellular Effects
Palmitoyl-CoA has significant effects on various types of cells and cellular processes. It influences cell function by participating in cell signaling pathways and affecting gene expression . For example, protein palmitoylation, which involves the attachment of palmitoyl groups from palmitoyl-CoA to proteins, is crucial for the proper function of many surface receptors and signaling proteins .
Molecular Mechanism
The mechanism of action of palmitoyl-CoA at the molecular level involves its binding interactions with biomolecules and its role in enzyme activation and changes in gene expression . For instance, palmitoyl-CoA is used as a substrate by palmitoyl acyltransferases, enzymes that catalyze the addition of palmitate to proteins . This modification can alter the function of the target protein, influencing cellular processes such as signal transduction.
Temporal Effects in Laboratory Settings
In laboratory settings, the effects of palmitoyl-CoA can change over time. For instance, the level of protein palmitoylation, a process involving palmitoyl-CoA, can fluctuate in response to various stimuli . This dynamic regulation allows for rapid adjustments in cellular functions.
Dosage Effects in Animal Models
The effects of palmitoyl-CoA can vary with different dosages in animal models. For instance, dysregulation of metabolic pathways involving palmitoyl-CoA has been linked to central nervous system disorders in experimental models .
Metabolic Pathways
Palmitoyl-CoA is involved in several metabolic pathways. It is a key metabolite in fatty acid metabolism, participating in both the synthesis and degradation of fatty acids . It also plays a role in the biosynthesis of sphingolipids .
Transport and Distribution
Palmitoyl-CoA can be transported into the mitochondrial matrix by the carnitine shuttle system, which transports fatty acyl-CoA molecules into the mitochondria . Once inside the mitochondria, palmitoyl-CoA can participate in beta-oxidation .
Subcellular Localization
The subcellular localization of palmitoyl-CoA is primarily within the mitochondria, where it participates in beta-oxidation . It can also be found in the cytosol, where it is involved in processes such as protein palmitoylation .
準備方法
合成経路および反応条件: パルミトイルCoAは、this compoundシンテターゼが触媒する反応によってパルミチン酸から合成されます。この反応は2段階のメカニズムで進行し、パルミトイル-AMPが中間体となります。全体的な反応は以下のとおりです: [ \text{パルミチン酸} + \text{CoA-SH} + \text{ATP} \rightarrow \text{パルミトイル-CoA} + \text{AMP} + \text{ピロリン酸} ] この反応は、ピロリン酸のexergonic加水分解によって完了まで駆動されます .
工業生産方法: this compoundの工業生産は、通常、this compoundシンテターゼを用いた酵素合成で行われます。このプロセスは、温度、pH、基質濃度などの反応条件を制御することで、収率と効率を最大限に高め、大規模生産に最適化されています .
反応の種類:
酸化: this compoundはミトコンドリア内でβ酸化を受け、クエン酸回路に進むアセチルCoAを生成します。
一般的な試薬と条件:
酸化: アシルCoAデヒドロゲナーゼなどの酵素と酸素の存在が必要です。
加水分解: 水とthis compoundヒドロラーゼが必要です.
主な生成物:
β酸化: アセチルCoAと還元された補酵素(NADHおよびFADH2)を生成します。
加水分解: 遊離パルミチン酸とCoAを生成します.
類似化合物との比較
Stearoyl coenzyme A: Another long-chain acyl-coenzyme A thioester, derived from stearic acid.
Myristoyl coenzyme A: Derived from myristic acid, involved in similar metabolic pathways.
Palmitoleoyl coenzyme A: Derived from palmitoleic acid, involved in lipid metabolism.
Uniqueness: Palmitoyl coenzyme A is unique due to its widespread role in both energy production through beta-oxidation and its involvement in protein palmitoylation, which regulates various cellular processes. Its dual functionality makes it a critical molecule in lipid metabolism and cellular signaling .
特性
IUPAC Name |
S-[2-[3-[[(2R)-4-[[[(2R,3S,4R,5R)-5-(6-aminopurin-9-yl)-4-hydroxy-3-phosphonooxyoxolan-2-yl]methoxy-hydroxyphosphoryl]oxy-hydroxyphosphoryl]oxy-2-hydroxy-3,3-dimethylbutanoyl]amino]propanoylamino]ethyl] hexadecanethioate | |
---|---|---|
Source | PubChem | |
URL | https://pubchem.ncbi.nlm.nih.gov | |
Description | Data deposited in or computed by PubChem | |
InChI |
InChI=1S/C37H66N7O17P3S/c1-4-5-6-7-8-9-10-11-12-13-14-15-16-17-28(46)65-21-20-39-27(45)18-19-40-35(49)32(48)37(2,3)23-58-64(55,56)61-63(53,54)57-22-26-31(60-62(50,51)52)30(47)36(59-26)44-25-43-29-33(38)41-24-42-34(29)44/h24-26,30-32,36,47-48H,4-23H2,1-3H3,(H,39,45)(H,40,49)(H,53,54)(H,55,56)(H2,38,41,42)(H2,50,51,52)/t26-,30-,31-,32+,36-/m1/s1 | |
Source | PubChem | |
URL | https://pubchem.ncbi.nlm.nih.gov | |
Description | Data deposited in or computed by PubChem | |
InChI Key |
MNBKLUUYKPBKDU-BBECNAHFSA-N | |
Source | PubChem | |
URL | https://pubchem.ncbi.nlm.nih.gov | |
Description | Data deposited in or computed by PubChem | |
Canonical SMILES |
CCCCCCCCCCCCCCCC(=O)SCCNC(=O)CCNC(=O)C(C(C)(C)COP(=O)(O)OP(=O)(O)OCC1C(C(C(O1)N2C=NC3=C(N=CN=C32)N)O)OP(=O)(O)O)O | |
Source | PubChem | |
URL | https://pubchem.ncbi.nlm.nih.gov | |
Description | Data deposited in or computed by PubChem | |
Isomeric SMILES |
CCCCCCCCCCCCCCCC(=O)SCCNC(=O)CCNC(=O)[C@@H](C(C)(C)COP(=O)(O)OP(=O)(O)OC[C@@H]1[C@H]([C@H]([C@@H](O1)N2C=NC3=C(N=CN=C32)N)O)OP(=O)(O)O)O | |
Source | PubChem | |
URL | https://pubchem.ncbi.nlm.nih.gov | |
Description | Data deposited in or computed by PubChem | |
Molecular Formula |
C37H66N7O17P3S | |
Source | PubChem | |
URL | https://pubchem.ncbi.nlm.nih.gov | |
Description | Data deposited in or computed by PubChem | |
DSSTOX Substance ID |
DTXSID701027137 | |
Record name | Palmitoyl coenzyme A | |
Source | EPA DSSTox | |
URL | https://comptox.epa.gov/dashboard/DTXSID701027137 | |
Description | DSSTox provides a high quality public chemistry resource for supporting improved predictive toxicology. | |
Molecular Weight |
1005.9 g/mol | |
Source | PubChem | |
URL | https://pubchem.ncbi.nlm.nih.gov | |
Description | Data deposited in or computed by PubChem | |
Physical Description |
Solid | |
Record name | Palmityl-CoA | |
Source | Human Metabolome Database (HMDB) | |
URL | http://www.hmdb.ca/metabolites/HMDB0001338 | |
Description | The Human Metabolome Database (HMDB) is a freely available electronic database containing detailed information about small molecule metabolites found in the human body. | |
Explanation | HMDB is offered to the public as a freely available resource. Use and re-distribution of the data, in whole or in part, for commercial purposes requires explicit permission of the authors and explicit acknowledgment of the source material (HMDB) and the original publication (see the HMDB citing page). We ask that users who download significant portions of the database cite the HMDB paper in any resulting publications. | |
CAS No. |
1763-10-6 | |
Record name | Palmitoyl CoA | |
Source | CAS Common Chemistry | |
URL | https://commonchemistry.cas.org/detail?cas_rn=1763-10-6 | |
Description | CAS Common Chemistry is an open community resource for accessing chemical information. Nearly 500,000 chemical substances from CAS REGISTRY cover areas of community interest, including common and frequently regulated chemicals, and those relevant to high school and undergraduate chemistry classes. This chemical information, curated by our expert scientists, is provided in alignment with our mission as a division of the American Chemical Society. | |
Explanation | The data from CAS Common Chemistry is provided under a CC-BY-NC 4.0 license, unless otherwise stated. | |
Record name | Palmitoyl coenzyme A | |
Source | EPA DSSTox | |
URL | https://comptox.epa.gov/dashboard/DTXSID701027137 | |
Description | DSSTox provides a high quality public chemistry resource for supporting improved predictive toxicology. | |
Record name | S-palmitoylcoenzyme A | |
Source | European Chemicals Agency (ECHA) | |
URL | https://echa.europa.eu/substance-information/-/substanceinfo/100.015.616 | |
Description | The European Chemicals Agency (ECHA) is an agency of the European Union which is the driving force among regulatory authorities in implementing the EU's groundbreaking chemicals legislation for the benefit of human health and the environment as well as for innovation and competitiveness. | |
Explanation | Use of the information, documents and data from the ECHA website is subject to the terms and conditions of this Legal Notice, and subject to other binding limitations provided for under applicable law, the information, documents and data made available on the ECHA website may be reproduced, distributed and/or used, totally or in part, for non-commercial purposes provided that ECHA is acknowledged as the source: "Source: European Chemicals Agency, http://echa.europa.eu/". Such acknowledgement must be included in each copy of the material. ECHA permits and encourages organisations and individuals to create links to the ECHA website under the following cumulative conditions: Links can only be made to webpages that provide a link to the Legal Notice page. | |
Record name | Palmityl-CoA | |
Source | Human Metabolome Database (HMDB) | |
URL | http://www.hmdb.ca/metabolites/HMDB0001338 | |
Description | The Human Metabolome Database (HMDB) is a freely available electronic database containing detailed information about small molecule metabolites found in the human body. | |
Explanation | HMDB is offered to the public as a freely available resource. Use and re-distribution of the data, in whole or in part, for commercial purposes requires explicit permission of the authors and explicit acknowledgment of the source material (HMDB) and the original publication (see the HMDB citing page). We ask that users who download significant portions of the database cite the HMDB paper in any resulting publications. | |
Retrosynthesis Analysis
AI-Powered Synthesis Planning: Our tool employs the Template_relevance Pistachio, Template_relevance Bkms_metabolic, Template_relevance Pistachio_ringbreaker, Template_relevance Reaxys, Template_relevance Reaxys_biocatalysis model, leveraging a vast database of chemical reactions to predict feasible synthetic routes.
One-Step Synthesis Focus: Specifically designed for one-step synthesis, it provides concise and direct routes for your target compounds, streamlining the synthesis process.
Accurate Predictions: Utilizing the extensive PISTACHIO, BKMS_METABOLIC, PISTACHIO_RINGBREAKER, REAXYS, REAXYS_BIOCATALYSIS database, our tool offers high-accuracy predictions, reflecting the latest in chemical research and data.
Strategy Settings
Precursor scoring | Relevance Heuristic |
---|---|
Min. plausibility | 0.01 |
Model | Template_relevance |
Template Set | Pistachio/Bkms_metabolic/Pistachio_ringbreaker/Reaxys/Reaxys_biocatalysis |
Top-N result to add to graph | 6 |
Feasible Synthetic Routes
ANone: Palmitoyl-CoA is a key regulator of carnitine palmitoyltransferase I (CPT I), the enzyme responsible for transporting long-chain fatty acids into the mitochondria for oxidation. [] Malonyl-CoA, an intermediate in fatty acid synthesis, is a potent inhibitor of CPT I. [] High levels of palmitoyl-CoA can compete with malonyl-CoA for binding to CPT I, thus promoting fatty acid oxidation. [] Additionally, palmitoyl-CoA can activate AMPK, which phosphorylates and inactivates acetyl-CoA carboxylase (ACC), the enzyme responsible for malonyl-CoA synthesis. [, ] This further reduces malonyl-CoA levels, ultimately favoring fatty acid oxidation. [, ]
ANone: Palmitoyl-CoA can inhibit phosphofructokinase-1 (PFK-1), a key regulatory enzyme in glycolysis. [] This inhibition appears to occur through both covalent and non-covalent interactions, and is influenced by the cellular energy status. [] By inhibiting PFK-1, palmitoyl-CoA can shift energy metabolism away from glucose utilization and towards fatty acid oxidation. []
ANone: Palmitoyl-CoA can inhibit GAPDH enzyme activity through covalent thioesterification at Cys-244. [] This modification has been shown to facilitate the translocation of GAPDH to lipid membranes. [] This mechanism may contribute to the insulin resistance and reduced glucose utilization observed in lipotoxic states. []
ANone: Palmitoyl-CoA can inhibit platelet function by acting as an antagonist at P2Y1 and P2Y12 receptors. [] It inhibits ADP-induced platelet shape change, Ca2+ mobilization, and P-selectin expression. [] This suggests that acyl-CoAs like palmitoyl-CoA may act as endogenous modulators of platelet function. []
ANone: Yes, palmitoyl-CoA can potentiate diacylglycerol-activated protein kinase C by significantly reducing the amount of phosphatidylserine required for enzyme activation. [] This effect was observed with both purified rat brain PKC and human platelet PKC. [] Interestingly, the acyl-CoA thioester of the carcinogenic peroxisome-proliferator ciprofibrate also showed a similar effect on PKC's phosphatidylserine requirement. []
ANone: The molecular formula of palmitoyl-CoA is C23H40N7O17P3S. Its molecular weight is 767.59 g/mol.
ANone: While specific spectroscopic data is not mentioned in the provided research, palmitoyl-CoA can be analyzed using techniques like mass spectrometry and UV-visible spectroscopy. [, ] Mass spectrometry helps in determining the mass-to-charge ratio of ions and is particularly useful for studying palmitoyl-CoA binding and modifications. [, ] UV-visible spectroscopy can be utilized to monitor enzyme kinetics involving palmitoyl-CoA due to its absorbance in the UV region. []
ANone: Palmitoyl-CoA hydrolase activity is present in various cellular compartments, including the cytosol, microsomes, and mitochondria. [, , ] This enzymatic activity can degrade palmitoyl-CoA, influencing its stability within the cell. [, , ] Specific conditions like temperature, pH, and the presence of stabilizing agents like glycerol can impact palmitoyl-CoA stability during experimental procedures. []
ANone: Palmitoyl-CoA is a key substrate for several enzymatic reactions involved in lipid metabolism. [, , , , ] It acts as the acyl donor in the synthesis of phosphatidic acid, a precursor to various phospholipids and diacylglycerols. [, , ] Palmitoyl-CoA is also a substrate for carnitine palmitoyltransferase I (CPT I), facilitating the transport of long-chain fatty acids into the mitochondria for beta-oxidation. [] This highlights its central role in both lipid anabolism and catabolism.
ANone: Palmitoyl-CoA is a substrate for hepatic microsomal long-chain fatty acid CoA ligases (LCLs), which catalyze the acyl-CoA conjugation of xenobiotic carboxylic acids. [] This conjugation process is an important detoxification mechanism for removing foreign compounds from the body. []
ANone: While the provided research doesn't delve into detailed computational studies on palmitoyl-CoA, techniques like molecular docking and molecular dynamics simulations can be employed to study its interactions with enzymes like CPT I, PKC, and GAPDH. These studies can provide insights into the binding affinities, binding modes, and the structural basis for the observed effects of palmitoyl-CoA on these enzymes.
ANone: The length of the acyl chain in acyl-CoAs significantly influences their inhibitory effect on platelet function. [] Compounds with saturated acyl groups containing 16-18 carbons, like palmitoyl-CoA (16:0), were found to be the most potent inhibitors of ADP-induced platelet aggregation. []
ANone: The inclusion of glycerol or palmitoyl-CoA itself in the buffer solution can stabilize palmitoyl-CoA hydrolase activity during preparation and storage. [] This suggests that maintaining an adequate concentration of palmitoyl-CoA or using glycerol as a stabilizing agent can help preserve its stability during in vitro studies. []
試験管内研究製品の免責事項と情報
BenchChemで提示されるすべての記事および製品情報は、情報提供を目的としています。BenchChemで購入可能な製品は、生体外研究のために特別に設計されています。生体外研究は、ラテン語の "in glass" に由来し、生物体の外で行われる実験を指します。これらの製品は医薬品または薬として分類されておらず、FDAから任何の医療状態、病気、または疾患の予防、治療、または治癒のために承認されていません。これらの製品を人間または動物に体内に導入する形態は、法律により厳格に禁止されています。これらのガイドラインに従うことは、研究と実験において法的および倫理的な基準の遵守を確実にするために重要です。