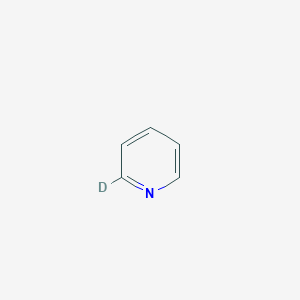
2-Deuteriopyridine
概要
説明
Pyridine-2-D1 is a deuterated derivative of pyridine, a six-membered heterocyclic aromatic compound. The incorporation of deuterium, a stable isotope of hydrogen, into the pyridine ring results in Pyridine-2-D1. This compound is widely used in various fields, including medical, environmental, and industrial research.
科学的研究の応用
Medicinal Chemistry Applications
Pyridine derivatives, including Pyridine-2-D1, are crucial in the development of various pharmaceutical compounds. The nitrogen atom in the pyridine ring contributes to the compound's basicity and solubility, making it an attractive scaffold for drug design.
Antimicrobial Activity
Recent studies have highlighted the effectiveness of pyridine derivatives against multidrug-resistant pathogens. For instance, certain pyridine compounds have been shown to inhibit the growth of Staphylococcus aureus (MRSA), showcasing their potential as novel antibiotics. The modification of pyridine scaffolds has led to the synthesis of various antimicrobial agents with improved efficacy and reduced side effects .
Anticancer Properties
Pyridine-2-D1 has been investigated for its anticancer properties. Research indicates that specific pyridine compounds can induce apoptosis and cell cycle arrest in cancer cells. For example, one study demonstrated that a novel pyridine compound exhibited significant cytotoxic activity against HepG2 liver cancer cells, with an IC50 value of 4.5 µM. The mechanism involved the upregulation of p53 and downregulation of cyclin D1, leading to G2/M phase arrest .
Neuroprotective Effects
Pyridine derivatives have also shown promise in neurology. Certain studies suggest that they may possess neuroprotective properties, making them candidates for treating neurodegenerative diseases like Alzheimer's. Their ability to interact with neurotransmitter systems could lead to advancements in neuropharmacology .
Chemical Synthesis Applications
Pyridine-2-D1 serves as an important reagent and solvent in synthetic organic chemistry. Its unique isotopic labeling allows for tracking reactions and understanding mechanisms at a molecular level.
Synthetic Pathways
The use of Pyridine-2-D1 in synthetic pathways has been documented extensively. For instance, it has been utilized in microwave-assisted synthesis techniques to create complex organic molecules efficiently. These methods often yield higher purity products and reduce reaction times significantly .
Catalysis
In addition to serving as a solvent, pyridine derivatives can act as ligands in catalytic processes. They are employed in various catalytic cycles, including those involving palladium-catalyzed reactions, which are essential for forming carbon-carbon bonds in organic synthesis .
Case Study 1: Anticancer Drug Development
A study focused on synthesizing new pyridine-based compounds aimed at targeting ovarian cancer cells. The synthesized compound showed enhanced potency compared to traditional chemotherapeutics like cisplatin, particularly against resistant cancer cell lines. This highlights the potential of Pyridine-2-D1 derivatives in overcoming drug resistance .
Case Study 2: Antimicrobial Research
Another significant study explored the antimicrobial efficacy of substituted pyridines against various bacterial strains. The results indicated that certain modifications to the pyridine ring could enhance antibacterial activity significantly, suggesting pathways for developing new antibiotics using Pyridine-2-D1 as a base structure .
作用機序
Target of Action
It’s worth noting that pyridine derivatives are known to interact with various biological targets, playing a significant role in numerous biochemical processes .
Mode of Action
For instance, dihydropyridines, a class of compounds that include pyridine derivatives, work by binding to and blocking voltage-gated L-type calcium channels found on smooth muscle cells of arterial blood vessels .
Biochemical Pathways
For example, they play a role in purine and pyrimidine metabolism . Additionally, microbial degradation of pyridine has been observed, suggesting involvement in microbial metabolic pathways .
Pharmacokinetics
Studies on similar compounds, such as 2’,2’-difluoro-2’-deoxycytidine (dfdc), have shown that these compounds can be rapidly inactivated by cytidine deaminase (cd) metabolism, affecting their bioavailability .
Result of Action
For instance, piperine, a piperidine derivative, shows powerful antioxidant action due to its capability of hindering or suppressing free radicals .
Action Environment
It’s known that environmental conditions can significantly impact the behavior and effectiveness of similar compounds, such as 2,4-dichlorophenoxyacetic acid (2,4-d), a commonly used herbicide .
準備方法
Synthetic Routes and Reaction Conditions: The synthesis of Pyridine-2-D1 typically involves the deuteration of pyridine. One common method is the exchange of hydrogen atoms in pyridine with deuterium using deuterium oxide (D2O) in the presence of a catalyst. This process can be facilitated by heating the reaction mixture under reflux conditions .
Industrial Production Methods: Industrial production of Pyridine-2-D1 often employs similar deuteration techniques but on a larger scale. The use of deuterium gas (D2) in the presence of a palladium catalyst is another method for achieving high levels of deuteration. This method ensures efficient and cost-effective production of Pyridine-2-D1 .
化学反応の分析
Types of Reactions: Pyridine-2-D1 undergoes various chemical reactions, including:
Oxidation: Oxidation of Pyridine-2-D1 can be achieved using oxidizing agents such as potassium permanganate or chromium trioxide.
Reduction: Reduction reactions can be carried out using reducing agents like lithium aluminum hydride or sodium borohydride.
Substitution: Electrophilic and nucleophilic substitution reactions are common, with reagents such as halogens, alkyl halides, and nucleophiles like amines and thiols.
Common Reagents and Conditions:
Oxidation: Potassium permanganate in acidic or basic medium.
Reduction: Lithium aluminum hydride in anhydrous ether.
Substitution: Halogens (chlorine, bromine) in the presence of a catalyst like iron(III) chloride.
Major Products:
Oxidation: Pyridine N-oxide derivatives.
Reduction: Deuterated piperidine derivatives.
Substitution: Various substituted pyridine derivatives depending on the substituent used.
類似化合物との比較
Pyridine: The non-deuterated parent compound of Pyridine-2-D1.
2-Fluoropyridine: A fluorinated derivative of pyridine with different electronic properties.
2-Chloropyridine: A chlorinated derivative with distinct reactivity patterns.
Uniqueness of Pyridine-2-D1: Pyridine-2-D1 is unique due to the incorporation of deuterium, which imparts different physical and chemical properties compared to its non-deuterated counterparts. The isotope effect can lead to changes in reaction rates, stability, and overall reactivity, making Pyridine-2-D1 a valuable tool in various scientific and industrial applications .
生物活性
Pyridine-2-D1 is a deuterated derivative of pyridine, a nitrogen-containing heterocyclic compound known for its diverse biological activities. This article explores the biological activity of Pyridine-2-D1, focusing on its pharmacological properties, mechanisms of action, and potential therapeutic applications based on recent research findings.
Overview of Pyridine Derivatives
Pyridine and its derivatives are recognized for their significant roles in medicinal chemistry. They exhibit a wide range of biological activities including antimicrobial, antiviral, anticancer, anti-inflammatory, and neuroprotective effects. The unique structural features of pyridine compounds contribute to their interactions with various biological targets, making them valuable in drug development.
1. Antimicrobial Activity
Recent studies have highlighted the antimicrobial potential of pyridine derivatives. Pyridine-2-D1 has been shown to possess significant antibacterial activity against various pathogens. For instance, compounds derived from pyridine exhibited minimum inhibitory concentrations (MIC) that suggest strong efficacy against Staphylococcus aureus and Escherichia coli .
Compound | Target Bacteria | MIC (µg/mL) |
---|---|---|
Pyridine-2-D1 | S. aureus | 56 ± 0.5 |
Pyridine-2-D1 | E. coli | 55 ± 0.5 |
2. Antiviral Activity
The antiviral properties of pyridine derivatives have gained attention, especially in the context of emerging viral infections such as COVID-19. Pyridine compounds have demonstrated activity against various viruses by inhibiting viral replication and enhancing host immune responses .
3. Anticancer Activity
Pyridine-2-D1 has shown promising results in cancer research. It has been evaluated for its antiproliferative effects on several cancer cell lines, including HeLa (cervical cancer) and MCF-7 (breast cancer). The compound's mechanism involves induction of apoptosis through reactive oxygen species (ROS) generation and cell cycle arrest at the G1 phase by inhibiting cyclin-dependent kinases (CDK4/6) .
Cell Line | IC50 (µM) | Mechanism of Action |
---|---|---|
HeLa | 28.3 | Apoptosis induction |
MCF-7 | 56.6 | ROS generation, CDK inhibition |
The biological activity of Pyridine-2-D1 can be attributed to several mechanisms:
- Apoptosis Induction : The compound triggers apoptosis in cancer cells through mitochondrial dysfunction and DNA damage .
- Cell Cycle Arrest : It inhibits key proteins involved in cell cycle progression, leading to halted proliferation .
- Antimicrobial Mechanism : The structural features of pyridine enhance binding to bacterial enzymes, disrupting vital metabolic pathways .
Case Study 1: Anticancer Efficacy
In a study assessing the anticancer properties of various pyridine derivatives, Pyridine-2-D1 was found to significantly reduce cell viability in MCF-7 cells compared to untreated controls. The study utilized MTT assays to quantify cell proliferation and confirmed apoptosis via DAPI staining methods.
Case Study 2: Antimicrobial Activity
Another investigation focused on the antimicrobial efficacy of Pyridine-2-D1 against clinical isolates of E. coli. The compound demonstrated a notable reduction in bacterial load in vitro, suggesting its potential as a therapeutic agent in treating bacterial infections resistant to conventional antibiotics.
特性
IUPAC Name |
2-deuteriopyridine | |
---|---|---|
Source | PubChem | |
URL | https://pubchem.ncbi.nlm.nih.gov | |
Description | Data deposited in or computed by PubChem | |
InChI |
InChI=1S/C5H5N/c1-2-4-6-5-3-1/h1-5H/i4D | |
Source | PubChem | |
URL | https://pubchem.ncbi.nlm.nih.gov | |
Description | Data deposited in or computed by PubChem | |
InChI Key |
JUJWROOIHBZHMG-QYKNYGDISA-N | |
Source | PubChem | |
URL | https://pubchem.ncbi.nlm.nih.gov | |
Description | Data deposited in or computed by PubChem | |
Canonical SMILES |
C1=CC=NC=C1 | |
Source | PubChem | |
URL | https://pubchem.ncbi.nlm.nih.gov | |
Description | Data deposited in or computed by PubChem | |
Isomeric SMILES |
[2H]C1=CC=CC=N1 | |
Source | PubChem | |
URL | https://pubchem.ncbi.nlm.nih.gov | |
Description | Data deposited in or computed by PubChem | |
Molecular Formula |
C5H5N | |
Source | PubChem | |
URL | https://pubchem.ncbi.nlm.nih.gov | |
Description | Data deposited in or computed by PubChem | |
Molecular Weight |
80.11 g/mol | |
Source | PubChem | |
URL | https://pubchem.ncbi.nlm.nih.gov | |
Description | Data deposited in or computed by PubChem | |
Retrosynthesis Analysis
AI-Powered Synthesis Planning: Our tool employs the Template_relevance Pistachio, Template_relevance Bkms_metabolic, Template_relevance Pistachio_ringbreaker, Template_relevance Reaxys, Template_relevance Reaxys_biocatalysis model, leveraging a vast database of chemical reactions to predict feasible synthetic routes.
One-Step Synthesis Focus: Specifically designed for one-step synthesis, it provides concise and direct routes for your target compounds, streamlining the synthesis process.
Accurate Predictions: Utilizing the extensive PISTACHIO, BKMS_METABOLIC, PISTACHIO_RINGBREAKER, REAXYS, REAXYS_BIOCATALYSIS database, our tool offers high-accuracy predictions, reflecting the latest in chemical research and data.
Strategy Settings
Precursor scoring | Relevance Heuristic |
---|---|
Min. plausibility | 0.01 |
Model | Template_relevance |
Template Set | Pistachio/Bkms_metabolic/Pistachio_ringbreaker/Reaxys/Reaxys_biocatalysis |
Top-N result to add to graph | 6 |
Feasible Synthetic Routes
Q1: Why was 2-deuteriopyridine used in this study on molybdenum oxide-alumina catalysts?
A: this compound was used as a probe molecule to study the nature of active sites on a molybdenum oxide-alumina catalyst. The researchers wanted to understand how pyridine interacts with the catalyst surface, specifically whether it interacts with acidic surface hydroxyl (OH) groups. By using this compound, where a deuterium atom replaces a hydrogen atom in the 2-position of the pyridine ring, they could track if the interaction with the surface resulted in deuterium exchange. The formation of this compound after adsorption confirmed that pyridine interacts with surface OD (hydroxyl groups where the hydrogen is replaced with deuterium) groups on the catalyst []. This interaction provides insights into the orientation of adsorbed pyridine and the nature of the active sites on the catalyst.
試験管内研究製品の免責事項と情報
BenchChemで提示されるすべての記事および製品情報は、情報提供を目的としています。BenchChemで購入可能な製品は、生体外研究のために特別に設計されています。生体外研究は、ラテン語の "in glass" に由来し、生物体の外で行われる実験を指します。これらの製品は医薬品または薬として分類されておらず、FDAから任何の医療状態、病気、または疾患の予防、治療、または治癒のために承認されていません。これらの製品を人間または動物に体内に導入する形態は、法律により厳格に禁止されています。これらのガイドラインに従うことは、研究と実験において法的および倫理的な基準の遵守を確実にするために重要です。