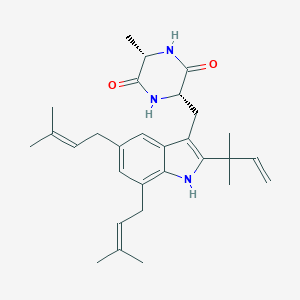
エキヌリン
概要
説明
エキヌリンは、1943年にアスペルギルス・エキヌラツスのカビから初めて単離された環状ジペプチドアルカロイドです . エキヌリンは、トリプレニル化インドール部分の特徴があり、アスペルギルス属やユーロチウム属など、さまざまな菌類で見られます . エキヌリンはさまざまな生物活性を示し、さまざまな科学分野で注目される化合物となっています .
製法
合成経路と反応条件
エキヌリンは、アスペルギルス・ルベールからのプレニル転移酵素、特にEchPT1とEchPT2の作用によって合成されます . これらの酵素は、シクロ-L-トリプトファン-L-アラニンの連続的なプレニル化を触媒し、トリプレニル化エキヌリンが主要生成物として生成されます . 反応条件は通常、ジメチルアリル二リン酸をプレニル供与体として使用することを伴います .
工業的生産方法
エキヌリンの工業的生産は、異種発現系によって達成できます。 たとえば、エキヌリン生産に関与する非リボソームペプチドシンテターゼをコードする遺伝子をクローニングし、アスペルギルス・オリゼーで発現させることができます . この方法により、エキヌリン関連の環状ジペプチドを効率的に生産することができます .
科学的研究の応用
Biological Activities of Echinulin
Echinulin exhibits a range of biological activities that make it a promising candidate for various therapeutic applications:
Antioxidant Activity
Echinulin demonstrates significant antioxidant properties, with an IC50 value of 18 µM, which is more potent than ascorbic acid (IC50 = 25 µM) . Its ability to scavenge free radicals positions it as a potential agent in preventing oxidative stress-related diseases.
Antimicrobial Properties
Echinulin has shown efficacy against various pathogens:
- Bacterial Activity: It exhibits antibacterial properties against Mycobacterium tuberculosis and other bacterial strains .
- Fungal Activity: Echinulin also displays antifungal activity, contributing to its potential use in treating fungal infections .
Neuroprotective Effects
Research indicates that echinulin derivatives can protect neuronal cells from damage induced by neurotoxins:
- Neuroprotection in Parkinson's Disease Models: (+)-Cryptoechinuline B, a derivative of echinulin, has been shown to increase cell viability in models of Parkinson's disease induced by neurotoxins such as rotenone and paraquat .
- Mechanism of Action: Echinulin may activate the NF-κB signaling pathway, enhancing T cell activation and potentially serving as an immunotherapeutic agent .
Anticancer Activity
Echinulin exhibits significant antiproliferative effects against various cancer cell lines:
- Colorectal Cancer: In studies involving HT-29 cells, echinulin demonstrated a potency nearly ten times greater than 5-Fluorouracil (5-FU), a standard treatment for colorectal cancer .
- Prostate Cancer: Echinulin was tested against three human prostate carcinoma cell lines (22Rv1, PC-3, LNCaP) with varying IC50 values indicating substantial cytotoxicity .
Comparative Analysis with Related Compounds
Echinulin belongs to a family of compounds known for similar biological activities. Below is a comparison table highlighting the activities of echinulin and its related compounds:
Compound | Antioxidant Activity | Antimicrobial Activity | Neuroprotective Activity | Anticancer Activity |
---|---|---|---|---|
Echinulin | High (IC50 = 18 µM) | Yes | Yes | Yes |
Neothis compound A | Moderate | Yes | Yes | Moderate |
Cryptoechinuline B | High | No | Yes | Low |
Variecolorin G | Moderate | Yes | No | Moderate |
Case Studies
Several studies have illustrated the potential applications of echinulin:
Neuroprotective Study
A study isolated (+)-cryptoechinuline B from Aspergillus niveoglaucus, demonstrating its protective effects in neuronal models subjected to oxidative stress. The compound significantly increased cell viability in various neurotoxin-induced models .
Anticancer Research
In vitro studies showed that echinulin induced apoptosis in HT-29 colorectal cancer cells by causing DNA fragmentation, outperforming traditional chemotherapeutics like irinotecan and 5-FU . This positions echinulin as a candidate for further investigation in cancer therapy.
作用機序
エキヌリンは、主に核因子-κB経路の活性化を介してその効果を発揮します . この経路は、免疫応答の調節に関与し、エキヌリンはこの経路を活性化することにより、さまざまな免疫機能を調節します . さらに、エキヌリンは一酸化窒素とプロスタグランジンE2の産生を阻害することが示されており、その抗炎症効果にさらに貢献しています .
類似化合物の比較
エキヌリンは、以下を含む、より大きなインドールジケトピペラジンアルカロイドファミリーの一部です。
ネオエキヌリンA: 類似の抗酸化作用と神経保護作用を示します.
クリプトthis compoundB: 神経保護作用で知られています.
バリエカラーリンG: 抗酸化作用を持つ、エキヌリン関連の別の化合物です.
これらの化合物と比較して、エキヌリンは、特定のトリプレニル化構造が特徴であり、これがその独特の生物活性を生み出しています .
生化学分析
Biochemical Properties
Echinulin contributes to the activation of T cell subsets, leading to the activation of the NF-κB pathway . This suggests that Echinulin interacts with T cells and the NF-κB pathway in its biochemical reactions. The nature of these interactions involves the modulation of T cell activity and the subsequent activation of the NF-κB pathway .
Cellular Effects
Echinulin has been found to exhibit neuroprotective activity in in vitro models of Parkinson’s disease . It protects neuronal cells against damage induced by paraquat and rotenone . This suggests that Echinulin influences cell function by protecting cells from damage and potentially impacting cell signaling pathways, gene expression, and cellular metabolism.
Molecular Mechanism
The molecular mechanism of Echinulin’s action involves its interaction with the NF-κB pathway . It contributes to the activation of T cell subsets, which leads to NF-κB activation . This suggests that Echinulin exerts its effects at the molecular level through binding interactions with biomolecules, potentially leading to enzyme activation and changes in gene expression.
Metabolic Pathways
Echinulin is a cyclic dipeptide formed through the condensation of two α-amino acids via non-ribosomal peptide synthetase (NRPS) This suggests that Echinulin is involved in the metabolic pathways related to the synthesis of cyclic dipeptides
準備方法
Synthetic Routes and Reaction Conditions
Echinulin is synthesized through the action of prenyltransferases, specifically EchPT1 and EchPT2, from Aspergillus ruber . These enzymes catalyze the consecutive prenylations of cyclo-l-tryptophan-l-alanine, leading to the formation of triprenylated echinulin as the predominant product . The reaction conditions typically involve the use of dimethylallyl diphosphate as the prenyl donor .
Industrial Production Methods
Industrial production of echinulin can be achieved through heterologous expression systems. For example, the gene encoding the non-ribosomal peptide synthetase responsible for echinulin production can be cloned and expressed in Aspergillus oryzae . This method allows for efficient production of echinulin-related cyclic dipeptides .
化学反応の分析
反応の種類
エキヌリンは、以下を含むさまざまな化学反応を起こします。
プレニル化: インドール環へのプレニル基の付加.
酸化: 分子へのヒドロキシル基の導入.
一般的な試薬と条件
生成される主な生成物
プレニル化: トリプレニル化エキヌリンは主な生成物です.
酸化: エキヌリンのヒドロキシル化誘導体.
科学研究での応用
エキヌリンとその誘導体は、さまざまな生物活性を調べられています。
抗酸化作用: エキヌリン関連化合物は、有意な抗酸化作用を示します.
抗菌作用: エキヌリンは抗菌作用と抗真菌作用を示しています.
類似化合物との比較
Echinulin is part of a larger family of indole diketopiperazine alkaloids, which include:
Neoechinulin A: Exhibits similar antioxidant and neuroprotective activities.
Cryptoechinuline B: Known for its neuroprotective properties.
Variecolorin G: Another echinulin-related compound with antioxidant activity.
Compared to these compounds, echinulin is unique due to its specific triprenylated structure, which contributes to its distinct biological activities .
生物活性
Echinulin, a compound derived from various fungi, has garnered attention for its diverse biological activities, including antimicrobial, cytotoxic, antiviral, and antioxidant properties. This article delves into the biological activity of echinulin, highlighting its mechanisms of action, efficacy in various cell lines, and potential therapeutic applications.
Overview of Echinulin
Echinulin is primarily isolated from species within the Aspergillus genus, particularly Aspergillus echinulatus and Aspergillus niveoglaucus. Recent studies have identified several derivatives of echinulin that exhibit significant biological activities, making them subjects of interest in pharmacological research.
Echinulin and its derivatives exert their biological effects through various mechanisms:
- Antiproliferative Activity : Echinulin has been shown to induce apoptosis in cancer cells. For instance, it causes DNA fragmentation in HT-29 colorectal cancer cells, demonstrating a significant increase in cell death compared to conventional chemotherapeutics like 5-fluorouracil (5-FU) and irinotecan .
- Neuroprotective Effects : Neoechinulin A, a derivative of echinulin, has demonstrated neuroprotective properties by safeguarding neuronal cells from oxidative stress induced by neurotoxins such as rotenone and paraquat . This suggests potential applications in treating neurodegenerative diseases.
- Antioxidant Properties : Echinulin exhibits strong antioxidant activity with an IC50 value lower than that of ascorbic acid, indicating its potential as a natural antioxidant agent .
Antiproliferative Activity
A comparative analysis of echinulin's antiproliferative effects against various cancer cell lines reveals compelling results:
Compound | Cell Line | IC50 (µM) | Comparison to 5-FU | Comparison to Irinotecan |
---|---|---|---|---|
Echinulin | HT-29 | 1.51 | 10x more active | 16x more active |
Neoechinulin A | Neuro-2a | Not tested | Not applicable | Not applicable |
8-Hydroxyechinulin | HT-29 | 30 | Less active | Less active |
Tardioxopiperazine B | B16 melanoma | 38.50 | Less active | Less active |
These findings indicate that echinulin is not only effective against colorectal cancer but also shows promise against other malignancies .
Neuroprotective Studies
Neoechinulins have been tested for their neuroprotective capabilities in models simulating Parkinson's disease:
- Neoechinulin A : Protects against MPP+ and rotenone-induced toxicity.
- Neoechinulin B : Exhibits cytoprotective activity in rotenone models.
- Cryptoechinuline B : Demonstrates significant viability enhancement in neurotoxin-treated cells .
Case Studies
- Colorectal Cancer Treatment : In a study comparing echinulin to traditional chemotherapeutics, echinulin showed superior efficacy in inducing apoptosis in HT-29 cells. The study highlighted the potential of echinulin as a novel therapeutic agent for chemoresistant colorectal cancer .
- Neurodegenerative Disease Models : Research involving Aspergillus niveoglaucus has shown that echinulin derivatives can significantly protect neuronal cells from oxidative damage, suggesting their use in developing treatments for diseases like Parkinson's .
特性
IUPAC Name |
(3S,6S)-3-methyl-6-[[2-(2-methylbut-3-en-2-yl)-5,7-bis(3-methylbut-2-enyl)-1H-indol-3-yl]methyl]piperazine-2,5-dione | |
---|---|---|
Source | PubChem | |
URL | https://pubchem.ncbi.nlm.nih.gov | |
Description | Data deposited in or computed by PubChem | |
InChI |
InChI=1S/C29H39N3O2/c1-9-29(7,8)26-23(16-24-28(34)30-19(6)27(33)31-24)22-15-20(12-10-17(2)3)14-21(25(22)32-26)13-11-18(4)5/h9-11,14-15,19,24,32H,1,12-13,16H2,2-8H3,(H,30,34)(H,31,33)/t19-,24-/m0/s1 | |
Source | PubChem | |
URL | https://pubchem.ncbi.nlm.nih.gov | |
Description | Data deposited in or computed by PubChem | |
InChI Key |
DIKMWTRJIZQJMY-CYFREDJKSA-N | |
Source | PubChem | |
URL | https://pubchem.ncbi.nlm.nih.gov | |
Description | Data deposited in or computed by PubChem | |
Canonical SMILES |
CC1C(=O)NC(C(=O)N1)CC2=C(NC3=C(C=C(C=C23)CC=C(C)C)CC=C(C)C)C(C)(C)C=C | |
Source | PubChem | |
URL | https://pubchem.ncbi.nlm.nih.gov | |
Description | Data deposited in or computed by PubChem | |
Isomeric SMILES |
C[C@H]1C(=O)N[C@H](C(=O)N1)CC2=C(NC3=C(C=C(C=C23)CC=C(C)C)CC=C(C)C)C(C)(C)C=C | |
Source | PubChem | |
URL | https://pubchem.ncbi.nlm.nih.gov | |
Description | Data deposited in or computed by PubChem | |
Molecular Formula |
C29H39N3O2 | |
Source | PubChem | |
URL | https://pubchem.ncbi.nlm.nih.gov | |
Description | Data deposited in or computed by PubChem | |
Molecular Weight |
461.6 g/mol | |
Source | PubChem | |
URL | https://pubchem.ncbi.nlm.nih.gov | |
Description | Data deposited in or computed by PubChem | |
CAS No. |
1859-87-6 | |
Record name | Echinuline | |
Source | ChemIDplus | |
URL | https://pubchem.ncbi.nlm.nih.gov/substance/?source=chemidplus&sourceid=0001859876 | |
Description | ChemIDplus is a free, web search system that provides access to the structure and nomenclature authority files used for the identification of chemical substances cited in National Library of Medicine (NLM) databases, including the TOXNET system. | |
Record name | ECHINULINE | |
Source | FDA Global Substance Registration System (GSRS) | |
URL | https://gsrs.ncats.nih.gov/ginas/app/beta/substances/3DP19VPN8U | |
Description | The FDA Global Substance Registration System (GSRS) enables the efficient and accurate exchange of information on what substances are in regulated products. Instead of relying on names, which vary across regulatory domains, countries, and regions, the GSRS knowledge base makes it possible for substances to be defined by standardized, scientific descriptions. | |
Explanation | Unless otherwise noted, the contents of the FDA website (www.fda.gov), both text and graphics, are not copyrighted. They are in the public domain and may be republished, reprinted and otherwise used freely by anyone without the need to obtain permission from FDA. Credit to the U.S. Food and Drug Administration as the source is appreciated but not required. | |
Retrosynthesis Analysis
AI-Powered Synthesis Planning: Our tool employs the Template_relevance Pistachio, Template_relevance Bkms_metabolic, Template_relevance Pistachio_ringbreaker, Template_relevance Reaxys, Template_relevance Reaxys_biocatalysis model, leveraging a vast database of chemical reactions to predict feasible synthetic routes.
One-Step Synthesis Focus: Specifically designed for one-step synthesis, it provides concise and direct routes for your target compounds, streamlining the synthesis process.
Accurate Predictions: Utilizing the extensive PISTACHIO, BKMS_METABOLIC, PISTACHIO_RINGBREAKER, REAXYS, REAXYS_BIOCATALYSIS database, our tool offers high-accuracy predictions, reflecting the latest in chemical research and data.
Strategy Settings
Precursor scoring | Relevance Heuristic |
---|---|
Min. plausibility | 0.01 |
Model | Template_relevance |
Template Set | Pistachio/Bkms_metabolic/Pistachio_ringbreaker/Reaxys/Reaxys_biocatalysis |
Top-N result to add to graph | 6 |
Feasible Synthetic Routes
Q1: What is the molecular formula and weight of echinulin?
A1: Echinulin possesses the molecular formula C29H39N3O2 and a molecular weight of 473.65 g/mol. []
Q2: What spectroscopic data is available for characterizing echinulin?
A2: Comprehensive 1H and 13C NMR spectral studies, including 1H-1H correlation, long-range 13C-1H correlation (HMBC and HMQC), and NOESY, have been employed to confirm the structure of echinulin and assign specific carbon and proton signals. Additionally, mass spectral analysis has been utilized for structural elucidation. []
Q3: How is echinulin biosynthesized?
A3: Echinulin biosynthesis involves a fascinating sequential prenylation cascade. The process begins with the prenylation of cyclo-L-alanyl-L-tryptophanyl. [] Two key enzymes, EchPT1 and EchPT2, govern this cascade in Aspergillus ruber. [, ] EchPT1 catalyzes the initial prenylation step, producing preechinulin. The remarkable EchPT2 then orchestrates the attachment of up to three dimethylallyl units to preechinulin and its dehydro forms, neoechinulins A and B. This results in the formation of a diverse array of at least 23 derivatives with varying prenylation patterns. []
Q4: What is the role of reverse prenylation in echinulin biosynthesis?
A4: Studies using labeled precursors have revealed that the aromatic isoprenylation at the 5- and 7-positions of the indole ring occurs with inversion of configuration at the allylic pyrophosphate, a characteristic of reverse prenylation. []
Q5: What is the significance of EchPT2 in echinulin biosynthesis?
A5: EchPT2 plays a unique role in generating structural diversity within the echinulin family. Its ability to catalyze consecutive prenylations on multiple substrates contributes to the wide array of echinulin derivatives observed. []
Q6: What biological activities have been reported for echinulin and its derivatives?
A6: Echinulin and its derivatives have demonstrated a range of biological activities, including:
- Antioxidant activity: Several echinulin derivatives, such as neoechinulin A, exhibit radical scavenging activity against DPPH radicals. [, , , ]
- Cytoprotective activity: Neoechinulin B has shown cytoprotective effects against rotenone-induced damage in a Parkinson's disease model. []
- Neuroprotective activity: (+)-Cryptoechinuline B, a specific enantiomer of cryptoechinuline B, displays neuroprotective properties in various in vitro Parkinson's disease models. []
- Immune modulation: Echinulin has been reported to activate T cells in humans, potentially through the NF-κB signaling pathway. []
- Antimycobacterial activity: Echinulin exhibited activity against Mycobacterium tuberculosis in vitro. []
Q7: Are there any potential applications of echinulin in drug discovery?
A7: The diverse biological activities of echinulin and its derivatives make them attractive candidates for drug discovery efforts. For instance, the neuroprotective effects of certain enantiomers like (+)-cryptoechinuline B warrant further investigation for potential therapeutic applications in neurodegenerative diseases like Parkinson's disease. []
Q8: Has echinulin shown potential as a SARS-CoV-2 Mpro inhibitor?
A8: Recent in vitro and in silico studies have identified neoechinulin A, an echinulin derivative, as a promising SARS-CoV-2 main protease (Mpro) inhibitor. It exhibited potent inhibitory activity against Mpro, comparable to the reference standard GC376. Molecular docking and molecular dynamics simulations provided insights into the potential binding mode and interactions of neoechinulin A with Mpro. []
Q9: What is known about the toxicity of echinulin?
A9: While echinulin exhibits various biological activities, it's crucial to acknowledge its potential toxicity. Studies have shown that echinulin can be toxic to rabbits. [, ] Additionally, mice exhibited aversion to water containing echinulin at certain concentrations. [] These findings underscore the need for careful assessment of the safety profile of echinulin and its derivatives before considering any potential therapeutic applications.
Q10: What analytical methods are employed to characterize and quantify echinulin?
A10: Various analytical techniques are used to study echinulin, including:* Chromatography: High-performance liquid chromatography (HPLC) coupled with mass spectrometry (MS) is commonly used for separation, identification, and quantification of echinulin and its derivatives in complex mixtures. [, , ] * Spectroscopy: Nuclear Magnetic Resonance (NMR) spectroscopy, including 1D and 2D techniques, plays a crucial role in elucidating the structure and stereochemistry of echinulin and its derivatives. []* Mass Spectrometry: High-resolution mass spectrometry (HRMS) provides accurate mass measurements, aiding in the identification and characterization of echinulin and its derivatives. [, ]
試験管内研究製品の免責事項と情報
BenchChemで提示されるすべての記事および製品情報は、情報提供を目的としています。BenchChemで購入可能な製品は、生体外研究のために特別に設計されています。生体外研究は、ラテン語の "in glass" に由来し、生物体の外で行われる実験を指します。これらの製品は医薬品または薬として分類されておらず、FDAから任何の医療状態、病気、または疾患の予防、治療、または治癒のために承認されていません。これらの製品を人間または動物に体内に導入する形態は、法律により厳格に禁止されています。これらのガイドラインに従うことは、研究と実験において法的および倫理的な基準の遵守を確実にするために重要です。