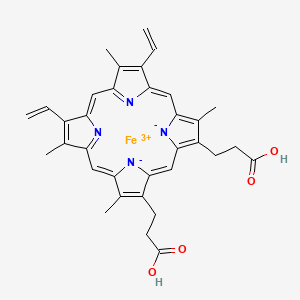
パンヘマチン
概要
説明
Hemin, also known as ferriprotoporphyrin IX chloride, is an iron-containing porphyrin compound. It is derived from heme, the iron-containing component of hemoglobin found in red blood cells. Hemin is characterized by the presence of a ferric iron (Fe³⁺) ion coordinated with a chloride ligand . This compound plays a crucial role in various biological processes and has significant pharmacological applications, particularly in the treatment of acute porphyria attacks .
科学的研究の応用
Hemin has a wide range of scientific research applications across various fields:
Chemistry: Hemin is used as a catalyst in organic synthesis and as a model compound for studying metalloporphyrins.
Biology: Hemin is utilized in studies related to heme metabolism and its role in cellular processes.
Industry: Hemin is employed in the production of heme-based products and as a component in diagnostic assays.
作用機序
Target of Action
Panhematin, also known as Hemin or Chlorohemin, is an iron-containing porphyrin . Its primary target is the enzyme δ-aminolevulinic acid synthase , which plays a crucial role in the synthesis of porphyrin in the liver and bone marrow .
Mode of Action
Panhematin acts by limiting the hepatic and/or marrow synthesis of porphyrin . This action is likely due to the inhibition of δ-aminolevulinic acid synthase , the rate-limiting enzyme in the porphyrin/heme biosynthetic pathway .
Biochemical Pathways
The biochemical pathway primarily affected by Panhematin is the heme biosynthetic pathway . By inhibiting δ-aminolevulinic acid synthase, Panhematin reduces the rate of porphyrin synthesis, thereby preventing the accumulation of neurotoxic porphyrin precursors .
Pharmacokinetics
The pharmacokinetics of Panhematin are best represented by a two-compartment model . After administration, hematin is first bound by hemopexin and, upon saturation, second by albumin . This results in a rapid initial clearance rate followed by a slower and prolonged second phase . The highest intravenous human hematin dose reported in the literature was 12.2 mg/kg, which resulted in acute gastrointestinal pain, paresthesia, and acute tubercular necrosis .
Result of Action
The administration of Panhematin leads to a decrease in the synthesis of porphyrin, reducing the accumulation of neurotoxic porphyrin precursors . This results in the amelioration of recurrent attacks of acute intermittent porphyria .
Action Environment
The action of Panhematin can be influenced by various environmental factors. For instance, the reconstitution of hematin with human serum albumin produces a significantly more stable preparation than reconstitution with sterile water, leading to a more tolerable administration with less hemostatic interference . Furthermore, the efficacy of Panhematin can be enhanced by an appropriate period of carbohydrate loading prior to administration .
生化学分析
Biochemical Properties
Panhematin works by lowering the production of a certain enzyme in the body . It has been shown to interact with various enzymes, proteins, and other biomolecules. For instance, it is known to inhibit the enzyme δ-aminolevulinic acid synthetase 1 (ALAS1) .
Cellular Effects
Panhematin has been observed to have significant effects on various types of cells and cellular processes . It helps control symptoms such as pain, increased heart rate or blood pressure, and changes in mental status .
Molecular Mechanism
The molecular mechanism of Panhematin involves its interaction with biomolecules and its role in enzyme inhibition or activation . It is protoporphyrin IX containing a ferric iron ion (heme B) with a chloride ligand .
Temporal Effects in Laboratory Settings
Panhematin undergoes rapid chemical decomposition in solution . Therefore, it should not be reconstituted until immediately before use .
Dosage Effects in Animal Models
While specific studies on the dosage effects of Panhematin in animal models are limited, the standard dose in clinical practice is 3 to 4 mg/kg/day . In more severe cases, the dose may be repeated no earlier than every 12 hours .
Metabolic Pathways
Panhematin is involved in the heme biosynthesis pathway . It limits the rate of porphyria/heme biosynthesis possibly by inhibiting the enzyme δ-aminolevulinic acid synthetase 1 (ALAS1) .
Transport and Distribution
Panhematin is administered intravenously . It is recommended to use a large arm vein or a central venous catheter for administration to minimize the risk of phlebitis .
準備方法
Synthetic Routes and Reaction Conditions: Hemin can be synthesized from hemoglobin through a series of chemical reactions. One common method involves heating hemoglobin with acetic acid and sodium chloride, which facilitates the conversion of heme to hemin . The reaction conditions typically include maintaining a specific temperature and pH to ensure the efficient formation of hemin.
Industrial Production Methods: Industrial production of hemin often involves the extraction of hemoglobin from animal blood, followed by its chemical conversion to hemin. The process includes purification steps to isolate hemin in its pure form. The final product is usually obtained as a dark blue to black powder .
化学反応の分析
Types of Reactions: Hemin undergoes various chemical reactions, including oxidation, reduction, and substitution. These reactions are influenced by the presence of different reagents and conditions.
Common Reagents and Conditions:
Substitution: Hemin can undergo substitution reactions where the chloride ligand is replaced by other ligands, such as hydroxide ions.
Major Products Formed:
Oxidation: Hematinic acid
Reduction: Heme
Substitution: Various heme derivatives depending on the substituent ligands
類似化合物との比較
- Hematin
- Heme
- Protoporphyrin IX
特性
CAS番号 |
16009-13-5 |
---|---|
分子式 |
C34H32FeN4O4+ |
分子量 |
616.5 g/mol |
IUPAC名 |
3-[18-(2-carboxyethyl)-8,13-bis(ethenyl)-3,7,12,17-tetramethylporphyrin-21,24-diid-2-yl]propanoic acid;iron(3+) |
InChI |
InChI=1S/C34H34N4O4.Fe/c1-7-21-17(3)25-13-26-19(5)23(9-11-33(39)40)31(37-26)16-32-24(10-12-34(41)42)20(6)28(38-32)15-30-22(8-2)18(4)27(36-30)14-29(21)35-25;/h7-8,13-16H,1-2,9-12H2,3-6H3,(H4,35,36,37,38,39,40,41,42);/q;+3/p-2 |
InChIキー |
GGIDWJQWCUJYRY-UHFFFAOYSA-L |
SMILES |
CC1=C(C2=CC3=C(C(=C([N-]3)C=C4C(=C(C(=N4)C=C5C(=C(C(=N5)C=C1[N-]2)C)C=C)C)C=C)C)CCC(=O)O)CCC(=O)O.[Cl-].[Fe+3] |
正規SMILES |
CC1=C(C2=CC3=C(C(=C([N-]3)C=C4C(=C(C(=N4)C=C5C(=C(C(=N5)C=C1[N-]2)C)C=C)C)C=C)C)CCC(=O)O)CCC(=O)O.[Fe+3] |
外観 |
Solid powder |
16009-13-5 | |
ピクトグラム |
Irritant; Health Hazard |
純度 |
>98% (or refer to the Certificate of Analysis) |
賞味期限 |
>2 years if stored properly |
溶解性 |
Soluble in DMSO |
保存方法 |
Dry, dark and at 0 - 4 C for short term (days to weeks) or -20 C for long term (months to years). |
同義語 |
Alkaline Hematin D 575 Alkaline Hematin D-575 Chloride, Ferriheme Chloride, Ferriprotoporphyrin IX Chlorohemin Ferrihaem Ferriheme Chloride Ferriprotoporphyrin Ferriprotoporphyrin IX Ferriprotoporphyrin IX Chloride Hematin Hematin D-575, Alkaline Hemin Panhematin Protohemin Protohemin IX |
製品の起源 |
United States |
Retrosynthesis Analysis
AI-Powered Synthesis Planning: Our tool employs the Template_relevance Pistachio, Template_relevance Bkms_metabolic, Template_relevance Pistachio_ringbreaker, Template_relevance Reaxys, Template_relevance Reaxys_biocatalysis model, leveraging a vast database of chemical reactions to predict feasible synthetic routes.
One-Step Synthesis Focus: Specifically designed for one-step synthesis, it provides concise and direct routes for your target compounds, streamlining the synthesis process.
Accurate Predictions: Utilizing the extensive PISTACHIO, BKMS_METABOLIC, PISTACHIO_RINGBREAKER, REAXYS, REAXYS_BIOCATALYSIS database, our tool offers high-accuracy predictions, reflecting the latest in chemical research and data.
Strategy Settings
Precursor scoring | Relevance Heuristic |
---|---|
Min. plausibility | 0.01 |
Model | Template_relevance |
Template Set | Pistachio/Bkms_metabolic/Pistachio_ringbreaker/Reaxys/Reaxys_biocatalysis |
Top-N result to add to graph | 6 |
Feasible Synthetic Routes
Q1: How does hemin interact with cells to influence erythroid progenitor cell growth?
A1: Hemin demonstrates a specific stimulatory effect on the growth of primitive murine erythroid progenitors (day 7 BFUE) in vitro []. This effect is dose-dependent and more pronounced in methylcellulose cultures compared to plasma clot cultures. Hemin's mechanism of action likely involves enhancing the proliferation and/or differentiation of these progenitor cells, as suggested by increased colony size and earlier appearance in culture.
Q2: Can hemin protect against hemin-induced neurotoxicity, and if so, how?
A2: While hemin is known to induce neurotoxicity, morphine has been shown to offer protection against this effect []. This protection is likely mediated by the activation of opioid receptors, leading to decreased intracellular calcium levels, a key player in hemin-induced neuronal death.
Q3: What role does hemin play in the context of sickle cell disease?
A3: Hemin exhibits a unique auto-amplification phenomenon in sickle cell disease, contributing to tissue injury []. Hemin infusion in sickle cell mice triggers acute intravascular hemolysis, leading to the release of even more hemin from red blood cells. This vicious cycle exacerbates oxidative stress and tissue damage.
Q4: How does hemin contribute to the virulence of Porphyromonas gingivalis, a periodontal pathogen?
A4: Hemin is essential for Porphyromonas gingivalis to survive in the iron-limited environment of the oral cavity []. Under iron-depleted conditions, the bacterium upregulates genes involved in iron uptake and host cell adhesion/invasion, processes crucial for its virulence.
Q5: Can hemin protect against cadmium stress in plants, and what are the underlying mechanisms?
A5: Hemin application has been shown to alleviate cadmium stress in maize by influencing sucrose and nitrogen metabolism, and regulating endogenous hormones []. Hemin enhances sucrose accumulation in leaves, promotes nitrogen uptake and assimilation, and modulates hormone levels, collectively contributing to improved plant tolerance against cadmium toxicity.
Q6: What is the molecular formula and weight of hemin?
A6: Hemin, also known as ferric chloride heme, has the molecular formula C34H32ClFeN4O4 and a molecular weight of 651.95 g/mol.
Q7: What spectroscopic techniques are used to characterize hemin and its interactions?
A7: Various spectroscopic methods have been employed to study hemin: * UV-Vis Spectroscopy: Used to monitor hemin binding to proteins and DNA, as well as its oxidation state changes [, , ]. * Fluorescence Spectroscopy: Utilized to study hemin interactions with lipoproteins [] and to detect zinc protoporphyrin IX uptake in cells []. * Nuclear Magnetic Resonance (NMR) Spectroscopy: Provides detailed structural information on hemin-protein complexes and their interactions with substrates [, ]. * Electron Paramagnetic Resonance (EPR) Spectroscopy: Used to identify and characterize radical species generated during hemin-catalyzed reactions []. * Resonance Raman Spectroscopy: Provides insights into the heme structure and environment in proteins and enzymes [].
Q8: Can hemin be incorporated into drug delivery systems?
A8: Hemin can be successfully incorporated into block copolymer micelles, creating artificial peroxidase systems with enhanced stability and catalytic activity in aqueous environments []. This highlights the potential of using hemin in diverse applications, including biocatalysis and biosensing.
Q9: Does hemin exhibit catalytic activity?
A9: Hemin demonstrates peroxidase-like activity, catalyzing the oxidation of various substrates in the presence of hydrogen peroxide [, ]. This property makes hemin-based systems attractive for applications such as biosensing and biocatalysis.
Q10: How does the structure of hemin relate to its ability to bind and oxidize lipoproteins?
A10: Hemin binds to lipoproteins like LDL and HDL with high affinity, even in the presence of other serum components []. The heme ring inserts into the lipoprotein surface monolayer, with the carboxylic acid groups interacting with positively charged regions of the protein. This binding facilitates the oxidation of lipoproteins by hemin in the presence of hydrogen peroxide, potentially contributing to atherosclerosis development.
Q11: Have computational methods been applied to study hemin and its interactions?
A11: Yes, computational studies, including molecular docking and molecular dynamics simulations, have provided valuable insights into hemin's interactions with proteins like the 2-oxoglutarate carrier []. These simulations help understand the binding modes and potential regulatory mechanisms of hemin on target proteins.
Q12: What is the impact of solid dispersion on hemin's pharmaceutical properties?
A12: Solid dispersion significantly improves the solubility and dissolution rate of hemin, enhancing its bioavailability by up to 49 times compared to pure hemin []. This formulation strategy holds promise for developing hemin-based therapeutics with improved efficacy.
Q13: How does hemin administration affect erythroid progenitor cells in a patient with acute variegate porphyria?
A13: Intravenous hemin treatment in a patient with acute variegate porphyria led to a decrease in the number of blood burst-forming unit-erythroid (BFU-E) cells []. This finding suggests that hemin can modulate erythropoiesis in vivo, highlighting its potential therapeutic application in erythropoietic disorders.
Q14: Has hemin's therapeutic potential been explored in animal models of disease?
A14: Yes, pre-treatment with hemin has shown protective effects against ischemia/reperfusion injury in rat hearts []. Hemin reduces infarct size, improves ventricular function, and decreases the release of cardiac injury markers. This protective effect is linked to the induction of heme oxygenase-1 (HO-1), an enzyme with antioxidant and anti-inflammatory properties.
Q15: Can topical hemin application improve wound healing in diabetic individuals?
A15: Topical application of hemin (0.5%) has demonstrated promising wound healing properties in a rat model of diabetes []. Hemin accelerates wound contraction, enhances collagen synthesis, and modulates the expression of pro- and anti-inflammatory cytokines, contributing to faster and more efficient wound repair.
Q16: Does hemin offer protection against high-fat diet-induced liver damage?
A16: Hemin treatment has been shown to protect against non-alcoholic steatohepatitis (NASH) induced by a high-fat diet in rats []. Hemin reduces liver enzyme levels (AST and ALT), decreases oxidative stress markers, and improves liver histology, suggesting its potential therapeutic application in NASH.
Q17: Can hemin be targeted to specific cell types?
A17: The development of hemin-loaded block copolymer micelles [] opens possibilities for targeted drug delivery. By modifying the surface properties of these micelles, it may be possible to achieve selective delivery of hemin to specific cell types or tissues, enhancing its therapeutic efficacy and minimizing off-target effects.
Q18: Are there any biomarkers to monitor hemin's therapeutic effects?
A18: Hemin induces the expression of HO-1, an enzyme with protective effects against oxidative stress and inflammation [, , ]. Therefore, HO-1 levels could potentially serve as a biomarker to monitor the therapeutic efficacy of hemin treatment.
Q19: How can hemin levels be measured in biological samples?
A19: Several analytical techniques are available to quantify hemin in biological samples. These include:* Spectrophotometry: Hemin absorbs light in the visible and ultraviolet regions, allowing for its quantification using spectrophotometric methods.* High-performance liquid chromatography (HPLC): This technique separates and quantifies hemin based on its chemical properties.
Q20: What factors influence the solubility of hemin?
A20: Hemin's solubility is influenced by several factors, including pH, temperature, and the presence of other molecules. Solid dispersion techniques have proven effective in enhancing hemin's solubility and dissolution rate [], improving its bioavailability for therapeutic applications.
試験管内研究製品の免責事項と情報
BenchChemで提示されるすべての記事および製品情報は、情報提供を目的としています。BenchChemで購入可能な製品は、生体外研究のために特別に設計されています。生体外研究は、ラテン語の "in glass" に由来し、生物体の外で行われる実験を指します。これらの製品は医薬品または薬として分類されておらず、FDAから任何の医療状態、病気、または疾患の予防、治療、または治癒のために承認されていません。これらの製品を人間または動物に体内に導入する形態は、法律により厳格に禁止されています。これらのガイドラインに従うことは、研究と実験において法的および倫理的な基準の遵守を確実にするために重要です。