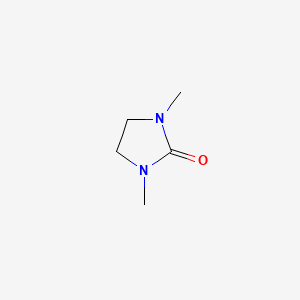
1,3-Dimethyl-2-imidazolidinone
概要
説明
1,3-Dimethyl-2-imidazolidinone (DMI) is a cyclic urea used as a high-boiling polar aprotic solvent . It is colorless and has high thermal and chemical stability . It is a homolog of the related solvent DMPU . DMI has excellent solvating ability for both inorganic and organic compounds .
Synthesis Analysis
DMI can be prepared from 1,2-dimethylethylenediamine by reaction with phosgene . It is reported to act as a promoter by minimizing the formation of dialkylation byproducts and accelerating the rate of monoalkylation of γ-butyrolactone .
Molecular Structure Analysis
The molecular formula of DMI is C5H10N2O . The molar mass is 114.1457 . The InChI key is CYSGHNMQYZDMIA-UHFFFAOYSA-N .
Chemical Reactions Analysis
DMI is reported to act as a promoter by minimizing the formation of dialkylation byproducts and accelerating the rate of monoalkylation of γ-butyrolactone . A first-principles density functional theory investigation on tailoring the fundamental reaction mechanism of synthesizing DMI through the urea method with water serving as both solvent and catalyst has been reported .
Physical and Chemical Properties Analysis
DMI is a colorless liquid . It has a melting point of 8.2°C and a boiling point of 225°C . The flash point is 120°C .
科学的研究の応用
Organic Synthesis and Catalysis
DMI has been demonstrated to be an effective promoter in the alkylation of γ-butyrolactone, significantly increasing the reaction rate and minimizing byproducts, with isolated yields of the monoalkylated product exceeding 90% (Li, Buzon, & Castaldi, 2001). It also plays a crucial role in atom transfer radical polymerizations (ATRPs) of methyl methacrylate, enhancing reaction rates and controllability, particularly in systems catalyzed by iron (Tian et al., 2018).
Improvement of Reaction Conditions
DMI is beneficial in the aromatic Finkelstein iodination (AFI) process, converting aryl bromides to aryl iodides. It accelerates the reaction rate and enables the production of products unattainable by previous methods, avoiding the use of more toxic solvents (Yamashita et al., 2012). In addition, DMI has been used to facilitate the formation of higher-order bromozincate in Negishi cross-coupling reactions, enhancing the reaction's efficiency (Hunter et al., 2011).
作用機序
Target of Action
1,3-Dimethyl-2-imidazolidinone (DMI) is a cyclic urea used as a high-boiling polar aprotic solvent . It is reported to act as a promoter by minimizing the formation of dialkylation byproducts and accelerating the rate of monoalkylation of γ-butyrolactone .
Mode of Action
DMI has excellent solvating ability for both inorganic and organic compounds . It is used in many applications, including detergents, dyestuffs, electronic materials, and in the manufacture of polymers . It can be prepared from 1,2-dimethylethylenediamine by reaction with phosgene .
Biochemical Pathways
The fundamental reaction mechanism of synthesizing DMI involves a nucleophilic cyclization reaction implemented by two ammonia removal steps . The role that water plays, besides as a solvent, more importantly, is to serve as a proton exchange bridge . This facilitates the proton migration by shortening the migration distance .
Pharmacokinetics
It is known that dmi has good chemical compatibility with metallic magnesium .
Result of Action
The result of DMI’s action is the promotion of various chemical reactions due to its strong polarity and chemical stability . It can dissolve many organic and inorganic compounds without reacting, making it an effective reaction solvent .
Action Environment
DMI has higher heat stability than general aprotic polar solvents in the presence of acids and alkalines . Its strong polarity and excellent solubility make it a versatile solvent in various environments . It is also known to have a high boiling point and thermal stability, making it suitable for use in high-temperature processes .
Safety and Hazards
将来の方向性
DMI is an excellent electrolyte solvent for Li–O2 batteries . It has higher Li2O2 and Li2CO3 solubility, which depresses cathode passivation during discharge, and promotes the liquid-phase redox shuttling during charge, consequently lowering the overpotential and improving the cyclability of the battery .
生化学分析
Biochemical Properties
DMI is reported to act as a promoter by minimizing the formation of dialkylation byproducts and accelerating the rate of monoalkylation of γ-butyrolactone . It exhibits high solubility in various inorganic and organic compounds due to its high dielectric constant and dipole moment .
Cellular Effects
It is also used as an excellent electrolyte solvent for Li–O2 batteries (LOBs), affecting the discharge-charge reaction kinetics and reversibility .
Molecular Mechanism
The molecular mechanism of DMI involves its role as a promoter in biochemical reactions. It minimizes the formation of dialkylation byproducts and accelerates the rate of monoalkylation of γ-butyrolactone . It can be prepared from 1,2-dimethylethylenediamine by reaction with phosgene .
Temporal Effects in Laboratory Settings
In laboratory settings, DMI has been shown to have high thermal and chemical stability . It is also reported to have higher Li2O2 and Li2CO3 solubility, which depresses cathode passivation during discharge and promotes the liquid-phase redox shuttling during charge, consequently lowering the overpotential and improving the cyclability of the battery .
Dosage Effects in Animal Models
It is known that DMI is toxic in contact with skin .
Metabolic Pathways
Dmi is reported to act as a promoter in biochemical reactions, specifically in the monoalkylation of γ-butyrolactone .
Transport and Distribution
DMI is a highly polar solvent and has excellent solvating ability for both inorganic and organic compounds . This suggests that it could be widely distributed within cells and tissues, interacting with various biomolecules.
Subcellular Localization
Given its solvating ability for both inorganic and organic compounds , it could potentially interact with various subcellular structures.
特性
IUPAC Name |
1,3-dimethylimidazolidin-2-one | |
---|---|---|
Source | PubChem | |
URL | https://pubchem.ncbi.nlm.nih.gov | |
Description | Data deposited in or computed by PubChem | |
InChI |
InChI=1S/C5H10N2O/c1-6-3-4-7(2)5(6)8/h3-4H2,1-2H3 | |
Source | PubChem | |
URL | https://pubchem.ncbi.nlm.nih.gov | |
Description | Data deposited in or computed by PubChem | |
InChI Key |
CYSGHNMQYZDMIA-UHFFFAOYSA-N | |
Source | PubChem | |
URL | https://pubchem.ncbi.nlm.nih.gov | |
Description | Data deposited in or computed by PubChem | |
Canonical SMILES |
CN1CCN(C1=O)C | |
Source | PubChem | |
URL | https://pubchem.ncbi.nlm.nih.gov | |
Description | Data deposited in or computed by PubChem | |
Molecular Formula |
C5H10N2O | |
Source | PubChem | |
URL | https://pubchem.ncbi.nlm.nih.gov | |
Description | Data deposited in or computed by PubChem | |
DSSTOX Substance ID |
DTXSID1073153 | |
Record name | 1,3-Dimethyl-2-imidazolidinone | |
Source | EPA DSSTox | |
URL | https://comptox.epa.gov/dashboard/DTXSID1073153 | |
Description | DSSTox provides a high quality public chemistry resource for supporting improved predictive toxicology. | |
Molecular Weight |
114.15 g/mol | |
Source | PubChem | |
URL | https://pubchem.ncbi.nlm.nih.gov | |
Description | Data deposited in or computed by PubChem | |
Physical Description |
Liquid | |
Record name | 2-Imidazolidinone, 1,3-dimethyl- | |
Source | EPA Chemicals under the TSCA | |
URL | https://www.epa.gov/chemicals-under-tsca | |
Description | EPA Chemicals under the Toxic Substances Control Act (TSCA) collection contains information on chemicals and their regulations under TSCA, including non-confidential content from the TSCA Chemical Substance Inventory and Chemical Data Reporting. | |
CAS No. |
80-73-9 | |
Record name | 1,3-Dimethyl-2-imidazolidinone | |
Source | CAS Common Chemistry | |
URL | https://commonchemistry.cas.org/detail?cas_rn=80-73-9 | |
Description | CAS Common Chemistry is an open community resource for accessing chemical information. Nearly 500,000 chemical substances from CAS REGISTRY cover areas of community interest, including common and frequently regulated chemicals, and those relevant to high school and undergraduate chemistry classes. This chemical information, curated by our expert scientists, is provided in alignment with our mission as a division of the American Chemical Society. | |
Explanation | The data from CAS Common Chemistry is provided under a CC-BY-NC 4.0 license, unless otherwise stated. | |
Record name | Dimethyl imidazolidinone | |
Source | ChemIDplus | |
URL | https://pubchem.ncbi.nlm.nih.gov/substance/?source=chemidplus&sourceid=0000080739 | |
Description | ChemIDplus is a free, web search system that provides access to the structure and nomenclature authority files used for the identification of chemical substances cited in National Library of Medicine (NLM) databases, including the TOXNET system. | |
Record name | 2-Imidazolidinone, 1,3-dimethyl- | |
Source | EPA Chemicals under the TSCA | |
URL | https://www.epa.gov/chemicals-under-tsca | |
Description | EPA Chemicals under the Toxic Substances Control Act (TSCA) collection contains information on chemicals and their regulations under TSCA, including non-confidential content from the TSCA Chemical Substance Inventory and Chemical Data Reporting. | |
Record name | 1,3-Dimethyl-2-imidazolidinone | |
Source | EPA DSSTox | |
URL | https://comptox.epa.gov/dashboard/DTXSID1073153 | |
Description | DSSTox provides a high quality public chemistry resource for supporting improved predictive toxicology. | |
Record name | 1,3-dimethylimidazolidin-2-one | |
Source | European Chemicals Agency (ECHA) | |
URL | https://echa.europa.eu/substance-information/-/substanceinfo/100.001.187 | |
Description | The European Chemicals Agency (ECHA) is an agency of the European Union which is the driving force among regulatory authorities in implementing the EU's groundbreaking chemicals legislation for the benefit of human health and the environment as well as for innovation and competitiveness. | |
Explanation | Use of the information, documents and data from the ECHA website is subject to the terms and conditions of this Legal Notice, and subject to other binding limitations provided for under applicable law, the information, documents and data made available on the ECHA website may be reproduced, distributed and/or used, totally or in part, for non-commercial purposes provided that ECHA is acknowledged as the source: "Source: European Chemicals Agency, http://echa.europa.eu/". Such acknowledgement must be included in each copy of the material. ECHA permits and encourages organisations and individuals to create links to the ECHA website under the following cumulative conditions: Links can only be made to webpages that provide a link to the Legal Notice page. | |
Record name | DIMETHYL IMIDAZOLIDINONE | |
Source | FDA Global Substance Registration System (GSRS) | |
URL | https://gsrs.ncats.nih.gov/ginas/app/beta/substances/5C1YQQ9M90 | |
Description | The FDA Global Substance Registration System (GSRS) enables the efficient and accurate exchange of information on what substances are in regulated products. Instead of relying on names, which vary across regulatory domains, countries, and regions, the GSRS knowledge base makes it possible for substances to be defined by standardized, scientific descriptions. | |
Explanation | Unless otherwise noted, the contents of the FDA website (www.fda.gov), both text and graphics, are not copyrighted. They are in the public domain and may be republished, reprinted and otherwise used freely by anyone without the need to obtain permission from FDA. Credit to the U.S. Food and Drug Administration as the source is appreciated but not required. | |
Retrosynthesis Analysis
AI-Powered Synthesis Planning: Our tool employs the Template_relevance Pistachio, Template_relevance Bkms_metabolic, Template_relevance Pistachio_ringbreaker, Template_relevance Reaxys, Template_relevance Reaxys_biocatalysis model, leveraging a vast database of chemical reactions to predict feasible synthetic routes.
One-Step Synthesis Focus: Specifically designed for one-step synthesis, it provides concise and direct routes for your target compounds, streamlining the synthesis process.
Accurate Predictions: Utilizing the extensive PISTACHIO, BKMS_METABOLIC, PISTACHIO_RINGBREAKER, REAXYS, REAXYS_BIOCATALYSIS database, our tool offers high-accuracy predictions, reflecting the latest in chemical research and data.
Strategy Settings
Precursor scoring | Relevance Heuristic |
---|---|
Min. plausibility | 0.01 |
Model | Template_relevance |
Template Set | Pistachio/Bkms_metabolic/Pistachio_ringbreaker/Reaxys/Reaxys_biocatalysis |
Top-N result to add to graph | 6 |
Feasible Synthetic Routes
ANone: 1,3-Dimethyl-2-imidazolidinone (DMI) has the molecular formula C5H10N2O and a molecular weight of 114.15 g/mol. []
ANone: DMI can be characterized using Infrared (IR) and Proton Nuclear Magnetic Resonance (1H NMR) spectroscopy. [, ] Additionally, Raman spectroscopy has been used to study the molecular deformation of DMI caused by hydrogen bonding. []
ANone: DMI was used as a cocrystal former with 1,3,5,7-tetranitro-1,3,5,7-tetrazocine (HMX), an explosive. Density Functional Theory (DFT-D) calculations revealed that the HMX/DMI cocrystal undergoes structural changes under hydrostatic pressure, becoming more metallic. []
ANone: Native Electrospray Ionization-Ion Mobility-Mass Spectrometry (ESI-IM-MS) studies demonstrated that low concentrations of DMI could improve the structural stability of apo-SOD1 by reducing average charge and dimer dissociation. []
ANone: DMI is completely miscible with water. [] Sweeping gas membrane distillation (SGMD) has been studied as a method for concentrating aqueous DMI solutions. []
ANone: While specific studies on the dissolution rate of DMI are limited in the provided research, factors like temperature, agitation, and the presence of other solutes can generally impact the dissolution rate of a compound.
ANone: Yes, DMI has been shown to be an effective solvent for various organic reactions, including aromatic Finkelstein iodination (AFI). [] It offers advantages over toxic solvents like N,N-dimethylformamide (DMF) and hexamethylphosphoramide.
ANone: DMI, when mixed with ionic liquids, forms organic electrolyte solutions (OES) capable of dissolving cellulose. [] These mixtures offer benefits like rapid dissolution, increased thermal stability, and reduced viscosity compared to pure ionic liquids.
ANone: DMI can be synthesized via the continuous chemical fixation of CO2 with N,N'-dimethylethylenediamine (DMEDA) using mesoporous silica catalysts like MCM-41 and HMS. In situ diffuse reflectance infrared Fourier transform (DRIFT) spectroscopy revealed mechanistic details of this reaction, highlighting the role of DMI in the process. [, ]
ANone: DMI has been investigated as an additive in lithium-ion battery electrolytes. [, ] It improves the performance by enhancing LiNO3 solubility, promoting stable SEI formation, and inhibiting LiPF6 decomposition.
ANone: Research indicates that a DMI-based electrolyte (LiBF4 in DMI/1,2-dimethoxyethane) can significantly enhance the discharge voltage of Li||CFx batteries without compromising capacity, suggesting potential for fast-charging applications. []
ANone: Headspace-selected ion flow tube mass spectrometry (HS-SIFT-MS) is a viable technique for analyzing residual DMI and other volatile impurities in pharmaceutical products. [] DMI, at concentrations up to 50% in water, is compatible with this method.
ANone: Size exclusion chromatography (SEC) coupled with multi-angle laser light scattering (MALLS) and quasi-elastic light scattering (QELS) detectors has been used to analyze DMI solutions containing cellulose and cellulose tricarbanilate. [] This technique helps study the molecular conformation and properties of cellulose in LiCl/DMI solutions.
ANone: Yes, gas-liquid chromatography has been successfully employed to determine the activity coefficients at infinite dilution of various solutes in DMI. [] This information provides insights into DMI's solvent properties and its potential for extractive distillation processes.
試験管内研究製品の免責事項と情報
BenchChemで提示されるすべての記事および製品情報は、情報提供を目的としています。BenchChemで購入可能な製品は、生体外研究のために特別に設計されています。生体外研究は、ラテン語の "in glass" に由来し、生物体の外で行われる実験を指します。これらの製品は医薬品または薬として分類されておらず、FDAから任何の医療状態、病気、または疾患の予防、治療、または治癒のために承認されていません。これらの製品を人間または動物に体内に導入する形態は、法律により厳格に禁止されています。これらのガイドラインに従うことは、研究と実験において法的および倫理的な基準の遵守を確実にするために重要です。