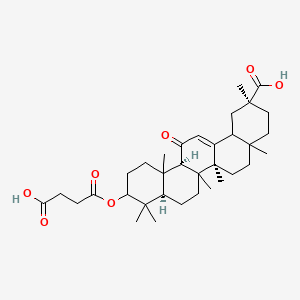
Carbenoxolone
概要
説明
Carbenoxolone (CBX) is a glycyrrhetinic acid derivative with a steroid-like structure, similar to substances found in the root of the licorice plant . It is used for the treatment of peptic, esophageal, and oral ulceration and inflammation .
Synthesis Analysis
Carbenoxolone is a semi-synthetic compound derived from glycyrrhizic acid (GZA), found in the root of licorice (Glycyrrhiza glabra) . Viral protein synthesis occurs in the presence of carbenoxolone but infectious virion formation is minimal, indicating that carbenoxolone blocks viral morphogenesis .Molecular Structure Analysis
Carbenoxolone has a complex molecular structure. Its IUPAC name is (3β)-3-[(3-carboxypropanoyl)oxy]-11-oxoolean-12-en-30-oic acid . The molecular formula is C34H50O7 and the molar mass is 570.767 g·mol−1 .Chemical Reactions Analysis
Carbenoxolone has been shown to hinder the replication of Vaccinia virus in a gap junction-independent manner in a human keratinocyte cell line . It also reversibly inhibits the conversion of inactive cortisone to cortisol by blocking 11β-hydroxysteroid dehydrogenase (11β-HSD) .Physical And Chemical Properties Analysis
Carbenoxolone is a small molecule with a chemical formula of C34H50O7 . It has a molar mass of 570.7566 .科学的研究の応用
Neuroprotection and Anti-Epileptic Applications
Carbenoxolone has been studied for its neuroprotective effects and potential as an anti-epileptic agent. It acts as a gap junction blocker, which can influence the synchronization of neuronal activity that is characteristic of epileptic seizures . Research suggests that by modulating gap junctions, carbenoxolone may help regulate potassium levels in astrocytes, affecting the formation of epileptic activity both in vitro and in vivo . This could have significant implications for developing new anti-epileptic therapies.
Material Science: Electrodeposition and Corrosion Protection
In material science, carbenoxolone serves as a multifunctional vehicle for the electrodeposition of materials such as carbon, polytetrafluoroethylene (PTFE), and their composites . Its amphiphilic structure allows it to adsorb on materials, enabling their charging and dispersion. Additionally, carbenoxolone exhibits film-forming properties, which are beneficial in creating protective coatings against corrosion .
Anti-Inflammatory Treatments
Carbenoxolone has been utilized for its anti-inflammatory properties. It is effective in treating peptic, esophageal, and oral ulceration and inflammation . Moreover, studies have shown that it can ameliorate allergic airway inflammation through the NF-κB/NLRP3 pathway in mice, suggesting potential applications in asthma treatment .
Cancer Research: Reducing Lung Metastases
Encapsulated carbenoxolone has been shown to reduce lung metastases in mice. Its antagonistic effect on high mobility group box 1 (HMGB1) and its ability to be delivered via a poly (lactic-co-glycolic acid) (PLGA) slow-release system for pulmonary delivery make it a promising candidate for cancer treatment, particularly in reducing the metastatic burden in the lungs .
Biosurfactant in Thin Film Deposition
Carbenoxolone’s role as a biosurfactant has been explored in the deposition of thin films. Its properties enable the electrophoretic deposition (EPD) of various materials, and it has been used to create films with specific microstructures and porosity, which are important in surface engineering strategies .
Epilepsy Research: Mechanisms and Therapeutics
The compound’s effects on epilepsy have been extensively researched, with studies focusing on its mechanism related to connexins and high-frequency oscillations. Carbenoxolone’s anticonvulsant effects are linked to its ability to interfere with the expression of connexins, particularly CX43, which may regulate the energy of pathological high-frequency oscillations and affect seizure formation .
作用機序
Target of Action
Carbenoxolone primarily targets two enzymes: 3-alpha- (or 20-beta)-hydroxysteroid dehydrogenase in Streptomyces exfoliatus and Corticosteroid 11-beta-dehydrogenase isozyme 1 in humans . These enzymes play crucial roles in the metabolism of steroids, which are involved in a variety of physiological processes, including inflammation and immune response.
Mode of Action
Carbenoxolone acts by inhibiting its target enzymes. Specifically, it reversibly inhibits the conversion of inactive cortisone to cortisol by blocking 11β-hydroxysteroid dehydrogenase (11β-HSD) . This inhibition disrupts the normal balance of corticosteroids in the body, leading to various effects.
Biochemical Pathways
Carbenoxolone affects several biochemical pathways. It has been found to reduce the expression of COX-2 , iNOS , and NF-κB , which are involved in inflammation and immune response . Additionally, it has been shown to induce heat shock proteins (HSPs), which help protect cells from stress .
Pharmacokinetics
It is known that the drug is orally active . More research is needed to fully understand its absorption, distribution, metabolism, and excretion (ADME) properties.
Result of Action
Carbenoxolone has several molecular and cellular effects. It has been found to decrease cell growth and viability in a time- and dose-dependent manner . It also reduces oxidative stress and shows anti-inflammatory effects in vivo . In the context of epilepsy, it has been shown to affect the formation of epileptic activity in astrocytes in vitro and epileptic seizure onset .
Action Environment
The action of Carbenoxolone can be influenced by environmental factors. For example, in the context of epilepsy, the astrocytic syncytium formed by gap junction-associated astrocytes, which is responsible for the regulation of potassium, affects the formation of epileptic activity in astrocytes in vitro and epileptic seizure onset . This suggests that the cellular environment can significantly influence the action, efficacy, and stability of Carbenoxolone.
Safety and Hazards
将来の方向性
Carbenoxolone has been identified as a potential inhibitor of FOXO3, a transcription factor associated with various tumor entities . It has also been found to reduce lung metastases in mice . These findings suggest that Carbenoxolone could be a promising candidate for the treatment of therapy-resistant high-stage neuroblastoma and other “FOXO-resistant” cancers .
特性
IUPAC Name |
(2S,4aS,6aR,6aS,6bR,8aR,10S,12aS,14bR)-10-(3-carboxypropanoyloxy)-2,4a,6a,6b,9,9,12a-heptamethyl-13-oxo-3,4,5,6,6a,7,8,8a,10,11,12,14b-dodecahydro-1H-picene-2-carboxylic acid | |
---|---|---|
Source | PubChem | |
URL | https://pubchem.ncbi.nlm.nih.gov | |
Description | Data deposited in or computed by PubChem | |
InChI |
InChI=1S/C34H50O7/c1-29(2)23-10-13-34(7)27(32(23,5)12-11-24(29)41-26(38)9-8-25(36)37)22(35)18-20-21-19-31(4,28(39)40)15-14-30(21,3)16-17-33(20,34)6/h18,21,23-24,27H,8-17,19H2,1-7H3,(H,36,37)(H,39,40)/t21-,23-,24-,27+,30+,31-,32-,33+,34+/m0/s1 | |
Source | PubChem | |
URL | https://pubchem.ncbi.nlm.nih.gov | |
Description | Data deposited in or computed by PubChem | |
InChI Key |
OBZHEBDUNPOCJG-WBXJDKIVSA-N | |
Source | PubChem | |
URL | https://pubchem.ncbi.nlm.nih.gov | |
Description | Data deposited in or computed by PubChem | |
Canonical SMILES |
CC1(C2CCC3(C(C2(CCC1OC(=O)CCC(=O)O)C)C(=O)C=C4C3(CCC5(C4CC(CC5)(C)C(=O)O)C)C)C)C | |
Source | PubChem | |
URL | https://pubchem.ncbi.nlm.nih.gov | |
Description | Data deposited in or computed by PubChem | |
Isomeric SMILES |
C[C@]12CC[C@](C[C@H]1C3=CC(=O)[C@@H]4[C@]5(CC[C@@H](C([C@@H]5CC[C@]4([C@@]3(CC2)C)C)(C)C)OC(=O)CCC(=O)O)C)(C)C(=O)O | |
Source | PubChem | |
URL | https://pubchem.ncbi.nlm.nih.gov | |
Description | Data deposited in or computed by PubChem | |
Molecular Formula |
C34H50O7 | |
Source | PubChem | |
URL | https://pubchem.ncbi.nlm.nih.gov | |
Description | Data deposited in or computed by PubChem | |
Related CAS |
7421-40-1 (di-hydrochloride salt) | |
Record name | Carbenoxolone [INN:BAN] | |
Source | ChemIDplus | |
URL | https://pubchem.ncbi.nlm.nih.gov/substance/?source=chemidplus&sourceid=0005697563 | |
Description | ChemIDplus is a free, web search system that provides access to the structure and nomenclature authority files used for the identification of chemical substances cited in National Library of Medicine (NLM) databases, including the TOXNET system. | |
DSSTOX Substance ID |
DTXSID4022733 | |
Record name | Carbenoxolone | |
Source | EPA DSSTox | |
URL | https://comptox.epa.gov/dashboard/DTXSID4022733 | |
Description | DSSTox provides a high quality public chemistry resource for supporting improved predictive toxicology. | |
Molecular Weight |
570.8 g/mol | |
Source | PubChem | |
URL | https://pubchem.ncbi.nlm.nih.gov | |
Description | Data deposited in or computed by PubChem | |
Product Name |
Carbenoxolone | |
CAS RN |
5697-56-3 | |
Record name | Carbenoxolone | |
Source | CAS Common Chemistry | |
URL | https://commonchemistry.cas.org/detail?cas_rn=5697-56-3 | |
Description | CAS Common Chemistry is an open community resource for accessing chemical information. Nearly 500,000 chemical substances from CAS REGISTRY cover areas of community interest, including common and frequently regulated chemicals, and those relevant to high school and undergraduate chemistry classes. This chemical information, curated by our expert scientists, is provided in alignment with our mission as a division of the American Chemical Society. | |
Explanation | The data from CAS Common Chemistry is provided under a CC-BY-NC 4.0 license, unless otherwise stated. | |
Record name | Carbenoxolone [INN:BAN] | |
Source | ChemIDplus | |
URL | https://pubchem.ncbi.nlm.nih.gov/substance/?source=chemidplus&sourceid=0005697563 | |
Description | ChemIDplus is a free, web search system that provides access to the structure and nomenclature authority files used for the identification of chemical substances cited in National Library of Medicine (NLM) databases, including the TOXNET system. | |
Record name | Carbenoxolone | |
Source | DrugBank | |
URL | https://www.drugbank.ca/drugs/DB02329 | |
Description | The DrugBank database is a unique bioinformatics and cheminformatics resource that combines detailed drug (i.e. chemical, pharmacological and pharmaceutical) data with comprehensive drug target (i.e. sequence, structure, and pathway) information. | |
Explanation | Creative Common's Attribution-NonCommercial 4.0 International License (http://creativecommons.org/licenses/by-nc/4.0/legalcode) | |
Record name | Olean-12-en-29-oic acid, 3-(3-carboxy-1-oxopropoxy)-11-oxo-, (3.beta.,20.beta.)- | |
Source | EPA Chemicals under the TSCA | |
URL | https://www.epa.gov/chemicals-under-tsca | |
Description | EPA Chemicals under the Toxic Substances Control Act (TSCA) collection contains information on chemicals and their regulations under TSCA, including non-confidential content from the TSCA Chemical Substance Inventory and Chemical Data Reporting. | |
Record name | Carbenoxolone | |
Source | EPA DSSTox | |
URL | https://comptox.epa.gov/dashboard/DTXSID4022733 | |
Description | DSSTox provides a high quality public chemistry resource for supporting improved predictive toxicology. | |
Record name | Carbenoxolone | |
Source | European Chemicals Agency (ECHA) | |
URL | https://echa.europa.eu/substance-information/-/substanceinfo/100.024.704 | |
Description | The European Chemicals Agency (ECHA) is an agency of the European Union which is the driving force among regulatory authorities in implementing the EU's groundbreaking chemicals legislation for the benefit of human health and the environment as well as for innovation and competitiveness. | |
Explanation | Use of the information, documents and data from the ECHA website is subject to the terms and conditions of this Legal Notice, and subject to other binding limitations provided for under applicable law, the information, documents and data made available on the ECHA website may be reproduced, distributed and/or used, totally or in part, for non-commercial purposes provided that ECHA is acknowledged as the source: "Source: European Chemicals Agency, http://echa.europa.eu/". Such acknowledgement must be included in each copy of the material. ECHA permits and encourages organisations and individuals to create links to the ECHA website under the following cumulative conditions: Links can only be made to webpages that provide a link to the Legal Notice page. | |
Record name | CARBENOXOLONE | |
Source | FDA Global Substance Registration System (GSRS) | |
URL | https://gsrs.ncats.nih.gov/ginas/app/beta/substances/MM6384NG73 | |
Description | The FDA Global Substance Registration System (GSRS) enables the efficient and accurate exchange of information on what substances are in regulated products. Instead of relying on names, which vary across regulatory domains, countries, and regions, the GSRS knowledge base makes it possible for substances to be defined by standardized, scientific descriptions. | |
Explanation | Unless otherwise noted, the contents of the FDA website (www.fda.gov), both text and graphics, are not copyrighted. They are in the public domain and may be republished, reprinted and otherwise used freely by anyone without the need to obtain permission from FDA. Credit to the U.S. Food and Drug Administration as the source is appreciated but not required. | |
Retrosynthesis Analysis
AI-Powered Synthesis Planning: Our tool employs the Template_relevance Pistachio, Template_relevance Bkms_metabolic, Template_relevance Pistachio_ringbreaker, Template_relevance Reaxys, Template_relevance Reaxys_biocatalysis model, leveraging a vast database of chemical reactions to predict feasible synthetic routes.
One-Step Synthesis Focus: Specifically designed for one-step synthesis, it provides concise and direct routes for your target compounds, streamlining the synthesis process.
Accurate Predictions: Utilizing the extensive PISTACHIO, BKMS_METABOLIC, PISTACHIO_RINGBREAKER, REAXYS, REAXYS_BIOCATALYSIS database, our tool offers high-accuracy predictions, reflecting the latest in chemical research and data.
Strategy Settings
Precursor scoring | Relevance Heuristic |
---|---|
Min. plausibility | 0.01 |
Model | Template_relevance |
Template Set | Pistachio/Bkms_metabolic/Pistachio_ringbreaker/Reaxys/Reaxys_biocatalysis |
Top-N result to add to graph | 6 |
Feasible Synthetic Routes
Q & A
Q1: What is Carbenoxolone’s primary mechanism of action?
A: Carbenoxolone is a known inhibitor of 11β-hydroxysteroid dehydrogenase (11β-HSD). [, , ] This enzyme complex interconverts cortisol and cortisone. [] By inhibiting 11β-HSD, carbenoxolone can alter glucocorticoid activity within cells. [, ]
Q2: How does carbenoxolone impact glucocorticoid activity?
A: Carbenoxolone inhibits both 11β-dehydrogenase and 11-oxoreductase, enzymes within the 11β-HSD complex. [] This dual inhibition distinguishes it from licorice, which primarily inhibits 11β-dehydrogenase. [] Carbenoxolone’s impact on glucocorticoid activity can vary depending on the tissue. In the kidney, it prevents cortisol from activating mineralocorticoid receptors. [, ] In the liver, it reduces intrahepatic cortisol concentrations, enhancing insulin sensitivity. []
Q3: Beyond 11β-HSD inhibition, does carbenoxolone interact with other targets?
A: Yes. Carbenoxolone has been shown to block gap junctions, specialized channels facilitating intercellular communication. [, , , , ] This blockage can influence various cellular processes, including neuronal signaling. [, , , ]
Q4: How does carbenoxolone affect gap junctions in the nervous system?
A: Research suggests that carbenoxolone blocks gap junctional intercellular communication, potentially influencing neuronal synchrony. [] Studies in rat models have demonstrated its anticonvulsant activity in both pentylenetetrazole (PTZ) and maximal electroshock (MES) seizure models. [] These findings suggest a potential role for gap junctions in epilepsy. [, , , ]
Q5: What is the molecular formula and weight of carbenoxolone?
A5: Carbenoxolone has the molecular formula C34H48O7 and a molecular weight of 572.7 g/mol.
Q6: Has carbenoxolone shown potential for material applications?
A: Research suggests carbenoxolone’s potential as a versatile biosurfactant in electrophoretic deposition (EPD) for creating films of various materials, including carbon materials, polytetrafluoroethylene (PTFE), and their composites. [] Its amphiphilic structure allows it to adsorb onto materials, enabling their charging and dispersion during EPD. []
A6: While carbenoxolone exhibits diverse biological activities, it is not typically recognized for possessing catalytic properties or being employed as a catalyst in chemical reactions.
A6: Currently, there's limited publicly available research exploring the application of computational chemistry and modeling techniques specifically to carbenoxolone.
A6: Further research is needed to establish a comprehensive understanding of the structure-activity relationship of carbenoxolone and its analogs.
A6: While carbenoxolone is available in various formulations, including tablets and capsules, specific details about its stability under various conditions and formulation strategies employed to enhance its stability, solubility, or bioavailability are not extensively documented in the provided research.
A6: Information about specific SHE (Safety, Health, and Environment) regulations pertaining to carbenoxolone is not explicitly covered in the provided research articles.
Q7: What is known about the absorption and distribution of carbenoxolone?
A: Carbenoxolone is readily absorbed after oral administration, achieving high plasma concentrations. [, ] It exhibits extensive binding to plasma proteins, which can influence its distribution and elimination. [, , ]
Q8: How is carbenoxolone metabolized and excreted?
A: Studies indicate that carbenoxolone is primarily excreted through the bile, with a smaller percentage eliminated in urine. [] In rats, a significant portion undergoes hydrolysis to enoxolone by stomach microflora. []
Q9: Does age affect carbenoxolone pharmacokinetics?
A: Research suggests age-related changes in carbenoxolone's plasma clearance and protein binding. [] Elderly individuals exhibit slower plasma clearance and reduced protein binding, potentially leading to higher levels of unbound, active drug. [] These age-related pharmacokinetic differences might contribute to an increased risk of adverse effects in older populations. []
Q10: What about its potential for other therapeutic applications?
A: Beyond its use in gastric ulcer treatment, research exploring carbenoxolone's therapeutic potential for conditions like cancer-induced bone pain [], epilepsy [, , , ], and even as a potential target for metabolic diseases like type 2 diabetes [, ] is ongoing.
A10: The provided research does not delve into specific resistance mechanisms or cross-resistance patterns associated with carbenoxolone.
A: While carbenoxolone has a history of use in ulcer treatment, it’s essential to acknowledge potential adverse effects. Research indicates it can lead to side effects such as fluid retention (edema), potassium loss (hypokalemia), and increased blood pressure, particularly in elderly individuals. [, , ]
A10: Specific strategies for targeted delivery of carbenoxolone to specific tissues or cells are not extensively discussed within the provided research.
A10: The research provided doesn't extensively explore the use of biomarkers for predicting carbenoxolone efficacy, monitoring treatment response, or identifying potential adverse effects.
Q11: What analytical methods are used to measure carbenoxolone concentrations?
A: Researchers have employed various techniques to analyze carbenoxolone, including spectrophotometry, [] gas chromatography, [] radioimmunoassay, [] and ultra-high-performance liquid chromatography tandem mass spectrometry (UHPLC-MS/MS). [] The choice of method depends on factors like the sample type (e.g., blood, brain tissue) and the desired sensitivity. []
A11: The research primarily focuses on carbenoxolone's pharmacological properties and doesn't extensively address its environmental impact or degradation pathways.
A11: Detailed studies on carbenoxolone's dissolution rate and solubility in various media are not provided in the research articles.
A11: While the research employs analytical methods for carbenoxolone quantification, specific details regarding method validation parameters like accuracy, precision, and specificity are not extensively elaborated upon.
A11: The provided research primarily focuses on carbenoxolone's pharmacological properties and doesn't delve into the specifics of quality control and assurance measures during its development, manufacturing, or distribution.
A11: Information concerning carbenoxolone's potential to elicit an immune response or strategies to mitigate immunogenicity is not extensively discussed in the research.
A11: The research primarily focuses on carbenoxolone's pharmacological properties and doesn't extensively address its potential interactions with drug transporters.
A11: Information regarding carbenoxolone's potential to induce or inhibit drug-metabolizing enzymes and strategies to manage these interactions is not extensively covered in the research.
A11: The research primarily focuses on carbenoxolone's pharmacological properties and doesn't extensively address its biocompatibility or biodegradability aspects.
A11: The research primarily focuses on carbenoxolone's pharmacological properties and doesn't extensively discuss alternative compounds or substitutes.
A11: The research primarily focuses on carbenoxolone's pharmacological properties and doesn't extensively address strategies for its recycling or waste management.
A11: The research primarily focuses on carbenoxolone's pharmacological properties and doesn't extensively discuss research infrastructure or resources related to the compound.
A: While the research highlights carbenoxolone's use as an ulcer-healing drug, [, , ] a comprehensive historical overview of its development and key research milestones is not provided.
A: The research showcases carbenoxolone's multidisciplinary nature, with studies spanning pharmacology, physiology, and cell biology. Its applications range from investigating fundamental cellular processes to exploring its potential in diverse therapeutic areas. [, ]
試験管内研究製品の免責事項と情報
BenchChemで提示されるすべての記事および製品情報は、情報提供を目的としています。BenchChemで購入可能な製品は、生体外研究のために特別に設計されています。生体外研究は、ラテン語の "in glass" に由来し、生物体の外で行われる実験を指します。これらの製品は医薬品または薬として分類されておらず、FDAから任何の医療状態、病気、または疾患の予防、治療、または治癒のために承認されていません。これらの製品を人間または動物に体内に導入する形態は、法律により厳格に禁止されています。これらのガイドラインに従うことは、研究と実験において法的および倫理的な基準の遵守を確実にするために重要です。