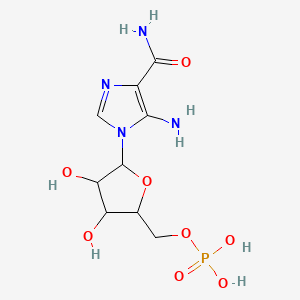
AICAR リボヌクレオチド
概要
説明
5-Aminoimidazole-4-carboxamide ribonucleotide is an intermediate in the generation of inosine monophosphate. It is an analog of adenosine monophosphate that is capable of stimulating AMP-dependent protein kinase activity . This compound has been studied for its potential therapeutic applications, including its use in treating diabetes and protecting against cardiac ischemic injury .
科学的研究の応用
5-Aminoimidazole-4-carboxamide ribonucleotide has a wide range of scientific research applications. It is commonly used as an activator of AMP-dependent protein kinase in studies related to metabolism, hypoxia, exercise, nucleotide synthesis, and cancer . Additionally, it has been shown to modulate microRNA expression in embryonic stem cells, highlighting its potential in regenerative medicine and organ transplantation .
作用機序
This inhibition enhances the rate of nucleotide re-synthesis, increasing adenosine generation from adenosine monophosphate during conditions of myocardial ischemia . In cardiac myocytes, acadesine is phosphorylated to 5-aminoimidazole-4-carboxamide ribonucleotide, which activates AMP-dependent protein kinase without changing the levels of nucleotides .
将来の方向性
AICA ribonucleotide has been used clinically to treat and protect against cardiac ischemic injury. The drug was first used in the 1980s as a method to preserve blood flow to the heart during surgery and is currently of interest as a potential treatment for diabetes by increasing the metabolic activity of tissues by changing the physical composition of muscle .
生化学分析
Biochemical Properties
AICA ribonucleotide plays a crucial role in biochemical reactions, particularly in the activation of AMP-dependent protein kinase. It interacts with various enzymes and proteins, including adenosine kinase and adenosine deaminase. These interactions enhance the rate of nucleotide re-synthesis and increase adenosine generation from adenosine monophosphate during myocardial ischemia . Additionally, AICA ribonucleotide can enter the de novo synthesis pathway for adenosine, inhibiting adenosine deaminase and causing an increase in ATP and adenosine levels .
Cellular Effects
AICA ribonucleotide influences various cellular processes, including cell signaling pathways, gene expression, and cellular metabolism. It has been shown to maintain the pluripotency of mouse embryonic stem cells by modulating protein expression . In human aortic endothelial cells, AICA ribonucleotide stimulates AMP-dependent protein kinase activity and nitric oxide production, which are crucial for vascular function . The compound also affects metabolic pathways, hypoxia response, and nucleotide synthesis, highlighting its broad impact on cellular function .
Molecular Mechanism
At the molecular level, AICA ribonucleotide exerts its effects through several mechanisms. It acts as an analog of adenosine, entering cardiac cells to inhibit adenosine kinase and adenosine deaminase . This inhibition enhances nucleotide re-synthesis and increases adenosine generation from adenosine monophosphate during myocardial ischemia . AICA ribonucleotide also activates AMP-dependent protein kinase without altering nucleotide levels, promoting various metabolic processes .
Temporal Effects in Laboratory Settings
In laboratory settings, the effects of AICA ribonucleotide can change over time. Studies have shown that the compound’s stability and degradation can influence its long-term effects on cellular function. For instance, AICA ribonucleotide has been used to maintain the pluripotency of mouse embryonic stem cells over extended periods . Additionally, its impact on nucleotide synthesis and metabolic pathways can vary depending on the duration of exposure .
Dosage Effects in Animal Models
The effects of AICA ribonucleotide vary with different dosages in animal models. At lower doses, the compound can stimulate AMP-dependent protein kinase activity and enhance metabolic processes without adverse effects . Higher doses may lead to toxic or adverse effects, including disruptions in nucleotide synthesis and cellular metabolism . These dosage-dependent effects highlight the importance of careful dosing in therapeutic applications.
Metabolic Pathways
AICA ribonucleotide is involved in several metabolic pathways, including the de novo synthesis of adenosine and the activation of AMP-dependent protein kinase . It interacts with enzymes such as adenosine kinase and adenosine deaminase, influencing metabolic flux and metabolite levels . The compound’s role in nucleotide synthesis and energy metabolism underscores its significance in cellular biochemistry .
Transport and Distribution
Within cells and tissues, AICA ribonucleotide is transported and distributed through various mechanisms. It can enter cardiac cells and other tissues, where it interacts with transporters and binding proteins . These interactions affect the compound’s localization and accumulation, influencing its overall activity and function .
Subcellular Localization
AICA ribonucleotide’s subcellular localization plays a critical role in its activity and function. The compound can be directed to specific compartments or organelles through targeting signals and post-translational modifications . This localization is essential for its interactions with enzymes and proteins, as well as its impact on cellular processes .
準備方法
Synthetic Routes and Reaction Conditions: The chemical synthesis of 5-aminoimidazole-4-carboxamide ribonucleotide involves multiple steps. One reliable method includes the use of adenine phosphoribosyltransferase from Escherichia coli to bind the nucleobase to the sugar moiety, followed by phosphorylation using polyphosphate kinases from Acinetobacter johnsonii and Meiothermus ruber . This multi-enzyme cascade allows for the production of diphosphorylated and triphosphorylated derivatives with high conversion rates .
Industrial Production Methods: Industrial production of 5-aminoimidazole-4-carboxamide ribonucleotide typically involves large-scale enzymatic synthesis due to the complexity and cost of chemical synthesis. The use of recombinant enzymes and optimized reaction conditions ensures high yield and purity of the final product .
化学反応の分析
Types of Reactions: 5-Aminoimidazole-4-carboxamide ribonucleotide undergoes various chemical reactions, including phosphorylation, dephosphorylation, and hydrolysis. These reactions are crucial for its biological activity and therapeutic applications .
Common Reagents and Conditions: Common reagents used in the synthesis and modification of 5-aminoimidazole-4-carboxamide ribonucleotide include phosphoribosyl pyrophosphate, adenine phosphoribosyltransferase, and polyphosphate kinases . The reactions are typically carried out under mild conditions to preserve the integrity of the compound.
Major Products: The major products formed from these reactions include diphosphorylated and triphosphorylated derivatives, which are essential for the compound’s biological activity .
類似化合物との比較
5-Aminoimidazole-4-carboxamide ribonucleotide is unique in its ability to activate AMP-dependent protein kinase and its potential therapeutic applications. Similar compounds include inosine monophosphate and nicotinamide riboside, both of which play roles in nucleotide metabolism and have distinct biological activities .
特性
IUPAC Name |
[5-(5-amino-4-carbamoylimidazol-1-yl)-3,4-dihydroxyoxolan-2-yl]methyl dihydrogen phosphate | |
---|---|---|
Details | Computed by Lexichem TK 2.7.0 (PubChem release 2021.05.07) | |
Source | PubChem | |
URL | https://pubchem.ncbi.nlm.nih.gov | |
Description | Data deposited in or computed by PubChem | |
InChI |
InChI=1S/C9H15N4O8P/c10-7-4(8(11)16)12-2-13(7)9-6(15)5(14)3(21-9)1-20-22(17,18)19/h2-3,5-6,9,14-15H,1,10H2,(H2,11,16)(H2,17,18,19) | |
Details | Computed by InChI 1.0.6 (PubChem release 2021.05.07) | |
Source | PubChem | |
URL | https://pubchem.ncbi.nlm.nih.gov | |
Description | Data deposited in or computed by PubChem | |
InChI Key |
NOTGFIUVDGNKRI-UHFFFAOYSA-N | |
Details | Computed by InChI 1.0.6 (PubChem release 2021.05.07) | |
Source | PubChem | |
URL | https://pubchem.ncbi.nlm.nih.gov | |
Description | Data deposited in or computed by PubChem | |
Canonical SMILES |
C1=NC(=C(N1C2C(C(C(O2)COP(=O)(O)O)O)O)N)C(=O)N | |
Details | Computed by OEChem 2.3.0 (PubChem release 2021.05.07) | |
Source | PubChem | |
URL | https://pubchem.ncbi.nlm.nih.gov | |
Description | Data deposited in or computed by PubChem | |
Molecular Formula |
C9H15N4O8P | |
Details | Computed by PubChem 2.1 (PubChem release 2021.05.07) | |
Source | PubChem | |
URL | https://pubchem.ncbi.nlm.nih.gov | |
Description | Data deposited in or computed by PubChem | |
DSSTOX Substance ID |
DTXSID50863081 | |
Record name | 5-Amino-1-(5-O-phosphonopentofuranosyl)-1H-imidazole-4-carboxamide | |
Source | EPA DSSTox | |
URL | https://comptox.epa.gov/dashboard/DTXSID50863081 | |
Description | DSSTox provides a high quality public chemistry resource for supporting improved predictive toxicology. | |
Molecular Weight |
338.21 g/mol | |
Details | Computed by PubChem 2.1 (PubChem release 2021.05.07) | |
Source | PubChem | |
URL | https://pubchem.ncbi.nlm.nih.gov | |
Description | Data deposited in or computed by PubChem | |
CAS RN |
3031-94-5 | |
Record name | AICAR | |
Source | DTP/NCI | |
URL | https://dtp.cancer.gov/dtpstandard/servlet/dwindex?searchtype=NSC&outputformat=html&searchlist=292227 | |
Description | The NCI Development Therapeutics Program (DTP) provides services and resources to the academic and private-sector research communities worldwide to facilitate the discovery and development of new cancer therapeutic agents. | |
Explanation | Unless otherwise indicated, all text within NCI products is free of copyright and may be reused without our permission. Credit the National Cancer Institute as the source. | |
Record name | AICAR | |
Source | DTP/NCI | |
URL | https://dtp.cancer.gov/dtpstandard/servlet/dwindex?searchtype=NSC&outputformat=html&searchlist=283955 | |
Description | The NCI Development Therapeutics Program (DTP) provides services and resources to the academic and private-sector research communities worldwide to facilitate the discovery and development of new cancer therapeutic agents. | |
Explanation | Unless otherwise indicated, all text within NCI products is free of copyright and may be reused without our permission. Credit the National Cancer Institute as the source. | |
Retrosynthesis Analysis
AI-Powered Synthesis Planning: Our tool employs the Template_relevance Pistachio, Template_relevance Bkms_metabolic, Template_relevance Pistachio_ringbreaker, Template_relevance Reaxys, Template_relevance Reaxys_biocatalysis model, leveraging a vast database of chemical reactions to predict feasible synthetic routes.
One-Step Synthesis Focus: Specifically designed for one-step synthesis, it provides concise and direct routes for your target compounds, streamlining the synthesis process.
Accurate Predictions: Utilizing the extensive PISTACHIO, BKMS_METABOLIC, PISTACHIO_RINGBREAKER, REAXYS, REAXYS_BIOCATALYSIS database, our tool offers high-accuracy predictions, reflecting the latest in chemical research and data.
Strategy Settings
Precursor scoring | Relevance Heuristic |
---|---|
Min. plausibility | 0.01 |
Model | Template_relevance |
Template Set | Pistachio/Bkms_metabolic/Pistachio_ringbreaker/Reaxys/Reaxys_biocatalysis |
Top-N result to add to graph | 6 |
Feasible Synthetic Routes
Q & A
Q1: How does AICA ribonucleotide interact with its targets, and what are the downstream effects?
A1: AICA ribonucleotide (AICAR) primarily acts as an AMP-activated protein kinase (AMPK) activator. [] AICAR mimics the effects of AMP, a key cellular energy sensor, leading to the activation of AMPK. This activation triggers a cascade of downstream effects, including:
- Upregulation of catabolic pathways: AMPK activation stimulates pathways that generate ATP, such as glucose uptake and fatty acid oxidation. []
- Downregulation of anabolic pathways: AMPK activation inhibits energy-consuming processes like protein synthesis and fatty acid synthesis. []
- Modulation of gene expression: AICAR treatment has been shown to influence the expression of genes involved in pluripotency, differentiation, and epigenetic modification. [, ]
Q2: What are the structural characteristics of AICA ribonucleotide?
A2: The molecular formula of AICA ribonucleotide is C9H15N4O8P. It has a molecular weight of 338.21 g/mol. Unfortunately, the provided research excerpts do not include specific details regarding spectroscopic data for AICA ribonucleotide.
Q3: Which enzymes are inhibited by AICA ribonucleotide in the de novo purine nucleotide biosynthesis pathway?
A3: Research suggests that AICA ribonucleotide primarily inhibits 5-aminoimidazole-4-carboxamide ribonucleotide formyltransferase (AICARFTase), the second folate-dependent enzyme in de novo purine biosynthesis. [, ] Some studies also indicate potential inhibitory effects on glycinamide ribonucleotide formyltransferase (GARFTase), although this inhibition is often secondary to AICARFTase inhibition. [, ]
Q4: How does the structure of AICA ribonucleotide analogues impact their activity and selectivity?
A4: Modifications to the AICA ribonucleotide structure, particularly in the bridge region and side chain, significantly influence its activity, potency, and selectivity. [, , ] For instance:
- Bridge length: Analogues with 4-carbon bridges exhibit higher potency toward FRα-expressing cells compared to those with shorter or longer bridges. [, ]
- Side chain modifications: Introducing aromatic substituents, fluorine atoms, or altering the bridge region's amide conformation can affect FRα selectivity and enzyme inhibition profiles. [, , ]
Q5: What is the role of folate receptors in the cellular uptake of AICA ribonucleotide and its analogues?
A5: AICA ribonucleotide analogues can be designed to utilize specific folate transporters for cellular entry. Compounds like AGF127 and AGF50 demonstrate selectivity for folate receptor alpha (FRα) over the reduced folate carrier (RFC) and the proton-coupled folate transporter (PCFT). [, ] This selectivity is crucial for targeted therapy, as FRα is overexpressed in many cancers but has limited expression in normal tissues. [, ]
Q6: How does AICA ribonucleotide influence autophagy in retinal pigment epithelial (RPE) cells?
A6: Studies show that AICAR induces autophagy in ARPE-19 cells, promoting the clearance of protein aggregates. [, ] This effect is particularly relevant in the context of age-related macular degeneration (AMD), where impaired protein degradation in RPE cells plays a significant role. [, ] AICAR's ability to induce autophagy and promote the removal of protein aggregates suggests its potential as a therapeutic target for AMD.
Q7: Are there any biomarkers associated with AICA ribonucleotide's mechanism of action or potential efficacy?
A7: While the research excerpts do not directly identify specific biomarkers for AICA ribonucleotide, they highlight its influence on several cellular processes and pathways that could be explored for potential biomarkers:
- AMPK activation: Measuring AMPK phosphorylation status could serve as a marker for AICAR activity. []
- ZMP accumulation: The intracellular accumulation of ZMP, the product of AICARFTase, could indicate AICAR's inhibitory effect on de novo purine biosynthesis. [, ]
- Gene expression changes: Monitoring the expression of genes involved in pluripotency, differentiation, or epigenetic modification, as influenced by AICAR, could provide insights into its effects on cellular function. [, ]
Q8: What are the potential applications of AICA ribonucleotide in cancer treatment?
A8: The research highlights several potential applications of AICA ribonucleotide and its analogues in cancer treatment:
- Targeting FRα-overexpressing tumors: Analogues selective for FRα could deliver therapeutic payloads specifically to cancer cells, minimizing off-target effects. [, ]
- Inhibiting de novo purine biosynthesis: Blocking AICARFTase and potentially GARFTase could disrupt purine nucleotide production, essential for cancer cell growth and proliferation. [, , ]
- Synergistic effects with existing therapies: AICAR's influence on AMPK and other signaling pathways might enhance the efficacy of existing cancer treatments. []
試験管内研究製品の免責事項と情報
BenchChemで提示されるすべての記事および製品情報は、情報提供を目的としています。BenchChemで購入可能な製品は、生体外研究のために特別に設計されています。生体外研究は、ラテン語の "in glass" に由来し、生物体の外で行われる実験を指します。これらの製品は医薬品または薬として分類されておらず、FDAから任何の医療状態、病気、または疾患の予防、治療、または治癒のために承認されていません。これらの製品を人間または動物に体内に導入する形態は、法律により厳格に禁止されています。これらのガイドラインに従うことは、研究と実験において法的および倫理的な基準の遵守を確実にするために重要です。