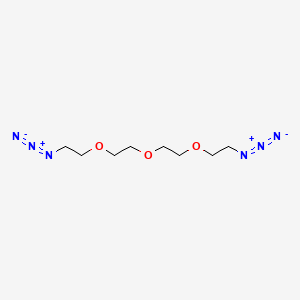
1,11-ジアジド-3,6,9-トリオキサウンデカン
概要
説明
1,11-Diazido-3,6,9-trioxaundecane is a homobifunctional polyethylene glycol azide compound. It is a liquid at room temperature and is known for its use in click chemistry as a cross-linking reagent. The compound has the molecular formula C8H16N6O3 and a molecular weight of 244.25 g/mol .
科学的研究の応用
1,11-Diazido-3,6,9-trioxaundecane has a wide range of applications in scientific research:
Chemistry: It is used as a cross-linking reagent in the synthesis of polymers and dendrimers. .
Biology: The compound is employed in bioconjugation techniques to link biomolecules such as proteins, nucleic acids, and carbohydrates. .
Medicine: In medicinal chemistry, 1,11-Diazido-3,6,9-trioxaundecane is used to create drug delivery systems and targeted therapies. .
Industry: The compound is utilized in the production of hydrogels, coatings, and adhesives. .
作用機序
Target of Action
The primary target of 1,11-Diazido-3,6,9-trioxaundecane is terminal alkynes and cyclooctyne derivatives . These targets are crucial in the formation of stable triazole linkages, which are essential in various biochemical reactions .
Mode of Action
1,11-Diazido-3,6,9-trioxaundecane interacts with its targets through a copper-catalyzed or strain-promoted 1,3-dipolar cycloaddition click reaction . This reaction results in the formation of a stable triazole linkage .
Pharmacokinetics
It is known that the compound is a liquid at room temperature , which may influence its absorption, distribution, metabolism, and excretion.
Result of Action
The primary result of the action of 1,11-Diazido-3,6,9-trioxaundecane is the formation of stable triazole linkages . These linkages can be used to synthesize various compounds, such as 1,11-bis[4-(pyren-1-ylmethoxymethyl)-1H-1,2,3-triazole-1-yl]-3,6,9-trioxaundecane, a fluorogenic chemosensor that can selectively detect Hg 2+ and Ag + ions in aqueous methanol solution .
Action Environment
The action, efficacy, and stability of 1,11-Diazido-3,6,9-trioxaundecane can be influenced by various environmental factors. Additionally, it should be stored at a temperature of -20°C to maintain its stability .
生化学分析
Biochemical Properties
1,11-Diazido-3,6,9-trioxaundecane is known to react via a copper-catalyzed or strain-promoted 1,3-dipolar cycloaddition click reaction with terminal alkynes and cyclooctyne derivatives to yield a stable triazole linkage . This property allows it to interact with a variety of biomolecules, particularly those containing alkyne or cyclooctyne groups.
Molecular Mechanism
The molecular mechanism of action of 1,11-Diazido-3,6,9-trioxaundecane primarily involves its ability to form stable triazole linkages. This is achieved through a copper-catalyzed or strain-promoted 1,3-dipolar cycloaddition click reaction with terminal alkynes and cyclooctyne derivatives .
Temporal Effects in Laboratory Settings
It is known to be a liquid at room temperature and has a density of 1.170 g/mL at 25 °C .
準備方法
Synthetic Routes and Reaction Conditions
1,11-Diazido-3,6,9-trioxaundecane can be synthesized through a multi-step process involving the reaction of polyethylene glycol with azide compounds. The general synthetic route involves the following steps:
Activation of Polyethylene Glycol: Polyethylene glycol is first activated by converting its terminal hydroxyl groups into tosylate or mesylate groups.
Substitution Reaction: The activated polyethylene glycol is then reacted with sodium azide in a polar aprotic solvent such as dimethylformamide (DMF) or dimethyl sulfoxide (DMSO) to replace the tosylate or mesylate groups with azide groups.
The reaction conditions typically involve heating the reaction mixture to around 80-100°C for several hours to ensure complete substitution .
Industrial Production Methods
Industrial production of 1,11-Diazido-3,6,9-trioxaundecane follows similar synthetic routes but on a larger scale. The process involves the use of large reactors and precise control of reaction conditions to ensure high yield and purity of the final product. The compound is then purified through distillation or chromatography techniques .
化学反応の分析
1,11-Diazido-3,6,9-trioxaundecane undergoes several types of chemical reactions, primarily involving its azide functional groups:
Click Chemistry: The azide groups react with terminal alkynes or cyclooctyne derivatives in the presence of a copper catalyst or strain-promoted conditions to form stable triazole linkages. .
Reduction: The azide groups can be reduced to amines using reducing agents such as lithium aluminum hydride (LiAlH4) or triphenylphosphine (PPh3) in the presence of water
The major products formed from these reactions include triazole-linked compounds and primary amines, respectively .
類似化合物との比較
1,11-Diazido-3,6,9-trioxaundecane can be compared with other similar compounds, such as:
1,17-Diazido-3,6,9,12,15-pentaoxaheptadecane: This compound has a longer polyethylene glycol chain and more azide groups, making it suitable for creating larger and more complex cross-linked structures
1,14-Diazido-3,6,9,12-tetraoxatetradecane: Similar to 1,11-Diazido-3,6,9-trioxaundecane but with a different chain length, affecting its solubility and reactivity
Polyethylene glycol bisazide: A related compound with multiple azide groups, used for similar applications in click chemistry and cross-linking
The uniqueness of 1,11-Diazido-3,6,9-trioxaundecane lies in its specific chain length and functional group arrangement, which provide a balance between solubility, reactivity, and stability .
特性
IUPAC Name |
1-azido-2-[2-[2-(2-azidoethoxy)ethoxy]ethoxy]ethane | |
---|---|---|
Source | PubChem | |
URL | https://pubchem.ncbi.nlm.nih.gov | |
Description | Data deposited in or computed by PubChem | |
InChI |
InChI=1S/C8H16N6O3/c9-13-11-1-3-15-5-7-17-8-6-16-4-2-12-14-10/h1-8H2 | |
Source | PubChem | |
URL | https://pubchem.ncbi.nlm.nih.gov | |
Description | Data deposited in or computed by PubChem | |
InChI Key |
SFMMXKLNFMIUCH-UHFFFAOYSA-N | |
Source | PubChem | |
URL | https://pubchem.ncbi.nlm.nih.gov | |
Description | Data deposited in or computed by PubChem | |
Canonical SMILES |
C(COCCOCCOCCN=[N+]=[N-])N=[N+]=[N-] | |
Source | PubChem | |
URL | https://pubchem.ncbi.nlm.nih.gov | |
Description | Data deposited in or computed by PubChem | |
Molecular Formula |
C8H16N6O3 | |
Source | PubChem | |
URL | https://pubchem.ncbi.nlm.nih.gov | |
Description | Data deposited in or computed by PubChem | |
DSSTOX Substance ID |
DTXSID10400052 | |
Record name | 1,11-DIAZIDO-3,6,9-TRIOXAUNDECANE | |
Source | EPA DSSTox | |
URL | https://comptox.epa.gov/dashboard/DTXSID10400052 | |
Description | DSSTox provides a high quality public chemistry resource for supporting improved predictive toxicology. | |
Molecular Weight |
244.25 g/mol | |
Source | PubChem | |
URL | https://pubchem.ncbi.nlm.nih.gov | |
Description | Data deposited in or computed by PubChem | |
CAS No. |
101187-39-7 | |
Record name | 1,11-DIAZIDO-3,6,9-TRIOXAUNDECANE | |
Source | EPA DSSTox | |
URL | https://comptox.epa.gov/dashboard/DTXSID10400052 | |
Description | DSSTox provides a high quality public chemistry resource for supporting improved predictive toxicology. | |
Record name | 1,11-Diazido-3,6,9-trioxaundecane | |
Source | European Chemicals Agency (ECHA) | |
URL | https://echa.europa.eu/information-on-chemicals | |
Description | The European Chemicals Agency (ECHA) is an agency of the European Union which is the driving force among regulatory authorities in implementing the EU's groundbreaking chemicals legislation for the benefit of human health and the environment as well as for innovation and competitiveness. | |
Explanation | Use of the information, documents and data from the ECHA website is subject to the terms and conditions of this Legal Notice, and subject to other binding limitations provided for under applicable law, the information, documents and data made available on the ECHA website may be reproduced, distributed and/or used, totally or in part, for non-commercial purposes provided that ECHA is acknowledged as the source: "Source: European Chemicals Agency, http://echa.europa.eu/". Such acknowledgement must be included in each copy of the material. ECHA permits and encourages organisations and individuals to create links to the ECHA website under the following cumulative conditions: Links can only be made to webpages that provide a link to the Legal Notice page. | |
Retrosynthesis Analysis
AI-Powered Synthesis Planning: Our tool employs the Template_relevance Pistachio, Template_relevance Bkms_metabolic, Template_relevance Pistachio_ringbreaker, Template_relevance Reaxys, Template_relevance Reaxys_biocatalysis model, leveraging a vast database of chemical reactions to predict feasible synthetic routes.
One-Step Synthesis Focus: Specifically designed for one-step synthesis, it provides concise and direct routes for your target compounds, streamlining the synthesis process.
Accurate Predictions: Utilizing the extensive PISTACHIO, BKMS_METABOLIC, PISTACHIO_RINGBREAKER, REAXYS, REAXYS_BIOCATALYSIS database, our tool offers high-accuracy predictions, reflecting the latest in chemical research and data.
Strategy Settings
Precursor scoring | Relevance Heuristic |
---|---|
Min. plausibility | 0.01 |
Model | Template_relevance |
Template Set | Pistachio/Bkms_metabolic/Pistachio_ringbreaker/Reaxys/Reaxys_biocatalysis |
Top-N result to add to graph | 6 |
Feasible Synthetic Routes
試験管内研究製品の免責事項と情報
BenchChemで提示されるすべての記事および製品情報は、情報提供を目的としています。BenchChemで購入可能な製品は、生体外研究のために特別に設計されています。生体外研究は、ラテン語の "in glass" に由来し、生物体の外で行われる実験を指します。これらの製品は医薬品または薬として分類されておらず、FDAから任何の医療状態、病気、または疾患の予防、治療、または治癒のために承認されていません。これらの製品を人間または動物に体内に導入する形態は、法律により厳格に禁止されています。これらのガイドラインに従うことは、研究と実験において法的および倫理的な基準の遵守を確実にするために重要です。