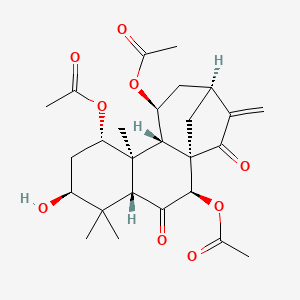
Adenanthin
説明
Adenanthin is a novel NF-κB and nucleophilic cysteines inhibitor. It has bacteriostatic, anti-inflammatory, and antitumor activities. Adenanthin also has antileukemic activity through targeting peroxiredoxin I/II .
Synthesis Analysis
Adenanthin is a diterpenoid isolated from the leaves of Isodon adenanthus . It has been reported to possess antileukemic activity through targeting peroxiredoxin I/II .Molecular Structure Analysis
The molecular formula of Adenanthin is C26H34O9 . The exact mass is 490.22 and the molecular weight is 490.550 .Chemical Reactions Analysis
Adenanthin has been reported to increase intracellular reactive oxygen species in leukemic and hepatocellular carcinoma cells . It inhibits adipogenesis of 3T3-L1 and mouse embryonic fibroblasts .Physical And Chemical Properties Analysis
The molecular weight of Adenanthin is 490.54 . The chemical formula is C26H34O9 .科学的研究の応用
Antileukemic Activity
Adenanthin has been reported to possess antileukemic activity . It targets peroxiredoxin I/II, which are enzymes involved in the body’s antioxidant defense system. This activity suggests potential therapeutic applications for leukemia treatment .
Obesity and Adipogenesis
Research indicates that Adenanthin may play a role in adipogenesis and the development of obesity. It has been shown to increase intracellular reactive oxygen species in cells, which could influence lipid accumulation and obesity-related pathways .
Antioxidant Enzyme Inhibition
Adenanthin serves as an inhibitor of thiol-dependent antioxidant enzymes. By inducing dysfunction of the PRDX-related antioxidant chain in NK cells, it helps to study the role of these antioxidants in greater detail .
作用機序
Biochemical Pathways
The increase in ROS due to the action of Adenanthin leads to the activation of extracellular signal–regulated kinases and increased transcription of CCAAT/enhancer-binding protein b . This contributes to adenanthin-induced differentiation of acute promyelocytic leukemia (APL) cells . In adipogenesis, adenanthin inhibits the RB-E2F1 signaling pathway and the expression and activity of C/EBPβ during mitotic clonal expansion .
Pharmacokinetics
Its therapeutic effects have been observed in experimental models .
Result of Action
Adenanthin has been reported to possess antileukemic activity . It induces differentiation of APL cells , represses tumor growth in vivo, and prolongs the survival of mouse APL models that are sensitive and resistant to retinoic acid . In adipogenesis, it inhibits the development of obesity by regulating ROS .
Action Environment
Its efficacy in experimental models suggests that it may be effective under a variety of conditions .
将来の方向性
特性
IUPAC Name |
[(1R,2R,4R,6S,8S,9S,10S,11S,13S)-2,8-diacetyloxy-6-hydroxy-5,5,9-trimethyl-14-methylidene-3,15-dioxo-11-tetracyclo[11.2.1.01,10.04,9]hexadecanyl] acetate | |
---|---|---|
Source | PubChem | |
URL | https://pubchem.ncbi.nlm.nih.gov | |
Description | Data deposited in or computed by PubChem | |
InChI |
InChI=1S/C26H34O9/c1-11-15-8-16(33-12(2)27)20-25(7)18(34-13(3)28)9-17(30)24(5,6)21(25)19(31)23(35-14(4)29)26(20,10-15)22(11)32/h15-18,20-21,23,30H,1,8-10H2,2-7H3/t15-,16+,17+,18+,20+,21-,23+,25+,26+/m1/s1 | |
Source | PubChem | |
URL | https://pubchem.ncbi.nlm.nih.gov | |
Description | Data deposited in or computed by PubChem | |
InChI Key |
WQVYSFSBBFDGRG-FYHXSELJSA-N | |
Source | PubChem | |
URL | https://pubchem.ncbi.nlm.nih.gov | |
Description | Data deposited in or computed by PubChem | |
Canonical SMILES |
CC(=O)OC1CC2CC3(C1C4(C(CC(C(C4C(=O)C3OC(=O)C)(C)C)O)OC(=O)C)C)C(=O)C2=C | |
Source | PubChem | |
URL | https://pubchem.ncbi.nlm.nih.gov | |
Description | Data deposited in or computed by PubChem | |
Isomeric SMILES |
CC(=O)O[C@H]1C[C@@H]2C[C@]3([C@@H]1[C@@]4([C@H](C[C@@H](C([C@H]4C(=O)[C@@H]3OC(=O)C)(C)C)O)OC(=O)C)C)C(=O)C2=C | |
Source | PubChem | |
URL | https://pubchem.ncbi.nlm.nih.gov | |
Description | Data deposited in or computed by PubChem | |
Molecular Formula |
C26H34O9 | |
Source | PubChem | |
URL | https://pubchem.ncbi.nlm.nih.gov | |
Description | Data deposited in or computed by PubChem | |
Molecular Weight |
490.5 g/mol | |
Source | PubChem | |
URL | https://pubchem.ncbi.nlm.nih.gov | |
Description | Data deposited in or computed by PubChem | |
Product Name |
Adenanthin |
Retrosynthesis Analysis
AI-Powered Synthesis Planning: Our tool employs the Template_relevance Pistachio, Template_relevance Bkms_metabolic, Template_relevance Pistachio_ringbreaker, Template_relevance Reaxys, Template_relevance Reaxys_biocatalysis model, leveraging a vast database of chemical reactions to predict feasible synthetic routes.
One-Step Synthesis Focus: Specifically designed for one-step synthesis, it provides concise and direct routes for your target compounds, streamlining the synthesis process.
Accurate Predictions: Utilizing the extensive PISTACHIO, BKMS_METABOLIC, PISTACHIO_RINGBREAKER, REAXYS, REAXYS_BIOCATALYSIS database, our tool offers high-accuracy predictions, reflecting the latest in chemical research and data.
Strategy Settings
Precursor scoring | Relevance Heuristic |
---|---|
Min. plausibility | 0.01 |
Model | Template_relevance |
Template Set | Pistachio/Bkms_metabolic/Pistachio_ringbreaker/Reaxys/Reaxys_biocatalysis |
Top-N result to add to graph | 6 |
Feasible Synthetic Routes
試験管内研究製品の免責事項と情報
BenchChemで提示されるすべての記事および製品情報は、情報提供を目的としています。BenchChemで購入可能な製品は、生体外研究のために特別に設計されています。生体外研究は、ラテン語の "in glass" に由来し、生物体の外で行われる実験を指します。これらの製品は医薬品または薬として分類されておらず、FDAから任何の医療状態、病気、または疾患の予防、治療、または治癒のために承認されていません。これらの製品を人間または動物に体内に導入する形態は、法律により厳格に禁止されています。これらのガイドラインに従うことは、研究と実験において法的および倫理的な基準の遵守を確実にするために重要です。