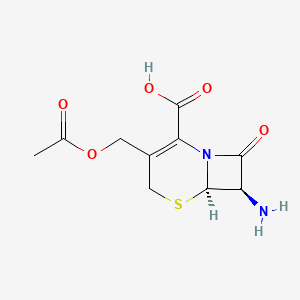
7-アミノセファロスポラン酸
概要
説明
7-アミノセファロスポラン酸は、セファロスポリン系抗生物質の合成に使用されるコア化学構造です。これは、Acremonium chrysogenumという菌類によって産生されるセファロスポリンCから誘導されます。 セファロスポリンは、ペニシリンと密接に関連するβ-ラクタム系抗生物質の大きなグループであり、さまざまな細菌感染症の治療に使用されます .
科学的研究の応用
7-Aminocephalosporanic acid is widely used in the pharmaceutical industry for the production of semi-synthetic cephalosporins. These antibiotics are crucial for treating bacterial infections, especially those caused by resistant strains . Additionally, 7-aminocephalosporanic acid derivatives have shown potential bioactivities, including enzyme inhibition .
作用機序
7-アミノセファロスポラン酸誘導体の作用機序には、細菌細胞壁の内側に位置するペニシリン結合タンパク質(PBP)への結合が含まれます。 この結合は、細菌細胞壁合成の第3段階および最終段階を阻害し、細胞溶解と死につながります . β-ラクタム環はこの活性に不可欠であり、PBPのD-アラニル-D-アラニン基質の構造を模倣しています .
類似の化合物:
6-アミノペニシラン酸: この化合物は、ペニシリン系抗生物質のコア構造です。
7-アミノデアセトキシセファロスポラン酸: セファロスポリン系抗生物質の合成に使用されるもう1つのコア構造.
独自性: 7-アミノセファロスポラン酸は、さまざまな活性スペクトルと耐性プロファイルを持つ、さまざまなセファロスポリン系抗生物質を形成する能力のためにユニークです。 そのβ-ラクタム環構造により、C3およびC7位置での修飾が可能になり、強化された特性を持つ抗生物質の開発につながります .
生化学分析
Biochemical Properties
7-ACA interacts with various enzymes and proteins. It contains a dihydro-thiazine ring and a β-lactam ring, which have a resistant effect on the class of Staphylococcus aureus penicillinase . The modifications of 7-ACA side-chains can lead to the alteration of pharmacokinetic properties and receptor binding affinity, thus creating a new class of cephalosporin antibiotics with important clinical uses .
Cellular Effects
7-ACA has significant effects on various types of cells and cellular processes. It is widely used for treating and preventing bacterial diseases by disrupting the synthesis of the peptidoglycan layer of cell walls in both gram-positive and gram-negative bacteria . The modifications of 7-ACA side-chains can affect the antibacterial activity and lead to alterations in cell function .
Molecular Mechanism
The mechanism of action of 7-ACA is primarily through its interactions with biomolecules. It exerts its effects at the molecular level, including binding interactions with biomolecules, enzyme inhibition or activation, and changes in gene expression . The modifications of the 7-ACA nucleus and substitutions on the side chains via semisynthetic means have produced differences among cephalosporins in antibacterial spectra, beta-lactamase sensitivities, and pharmacokinetics .
Temporal Effects in Laboratory Settings
Over time, the effects of 7-ACA can change in laboratory settings. Information on the product’s stability, degradation, and any long-term effects on cellular function observed in in vitro or in vivo studies is crucial. The production level of 7-ACA is nearly equivalent to the total β-lactams biosynthesized by the parental overproducing strain .
Metabolic Pathways
7-ACA is involved in the metabolic pathways of cephalosporin antibiotics. It is usually produced from cephalosporin-C (CPC) by the enzyme cephalosporin acylases . These enzymes are the groups of β-lactam acylases, highly specialized peptidases that are capable of cleaving the amide bond between a β-lactam nucleus and a side chain without damaging the β-lactam ring .
Subcellular Localization
Given that 7-ACA is a key intermediate in the production of semisynthetic cephalosporins, it is likely involved in cellular processes in specific compartments or organelles related to antibiotic synthesis .
準備方法
合成経路と反応条件: 7-アミノセファロスポラン酸は、セファロスポリンCの化学酵素的加水分解によって得られます。 このプロセスには、セファロスポリンアシラーゼ酵素の使用が含まれ、β-ラクタム環を損傷することなく、β-ラクタム核と側鎖の間のアミド結合を切断します .
工業的生産方法: 7-アミノセファロスポラン酸の工業的生産は、通常、Acremonium chrysogenumの発酵によってセファロスポリンCを生成し、続いて酵素的変換によって7-アミノセファロスポラン酸を生成することによって行われます。 このプロセスは、1段階または2段階の酵素的変換で行うことができます .
化学反応の分析
反応の種類: 7-アミノセファロスポラン酸は、アシル化、酸化、置換など、さまざまな化学反応を起こします。 これらの反応は、さまざまなセファロスポリン系抗生物質の合成に不可欠です .
一般的な試薬と条件:
アシル化: この反応には、ベンゾイルクロリドやシンナモイルクロリドなどのアシル化剤の使用が含まれます。
酸化: m-クロロ過安息香酸などの酸化剤を使用して、7-アミノセファロスポラン酸を酸化できます.
置換: さまざまな置換基を、β-ラクタム核のC3およびC7位置に導入して、さまざまなセファロスポリン誘導体を作成できます.
主な生成物: これらの反応から生成される主な生成物は、セフォタキシムやセフポドキシムプロキセチルなどのさまざまなセファロスポリン系抗生物質です .
4. 科学研究の用途
7-アミノセファロスポラン酸は、半合成セファロスポリンの生産のために製薬業界で広く使用されています。 これらの抗生物質は、特に耐性株によって引き起こされる細菌感染症の治療に不可欠です . さらに、7-アミノセファロスポラン酸誘導体は、酵素阻害など、潜在的な生物活性を示しています .
類似化合物との比較
6-Aminopenicillanic acid: This compound is the core structure for penicillin antibiotics.
7-Aminodeacetoxycephalosporanic acid: Another core structure used in the synthesis of cephalosporin antibiotics.
Uniqueness: 7-Aminocephalosporanic acid is unique due to its ability to form a wide variety of cephalosporin antibiotics with different spectra of activity and resistance profiles. Its β-lactam ring structure allows for modifications at the C3 and C7 positions, leading to the development of antibiotics with enhanced properties .
特性
IUPAC Name |
(6R,7R)-3-(acetyloxymethyl)-7-amino-8-oxo-5-thia-1-azabicyclo[4.2.0]oct-2-ene-2-carboxylic acid | |
---|---|---|
Source | PubChem | |
URL | https://pubchem.ncbi.nlm.nih.gov | |
Description | Data deposited in or computed by PubChem | |
InChI |
InChI=1S/C10H12N2O5S/c1-4(13)17-2-5-3-18-9-6(11)8(14)12(9)7(5)10(15)16/h6,9H,2-3,11H2,1H3,(H,15,16)/t6-,9-/m1/s1 | |
Source | PubChem | |
URL | https://pubchem.ncbi.nlm.nih.gov | |
Description | Data deposited in or computed by PubChem | |
InChI Key |
HSHGZXNAXBPPDL-HZGVNTEJSA-N | |
Source | PubChem | |
URL | https://pubchem.ncbi.nlm.nih.gov | |
Description | Data deposited in or computed by PubChem | |
Canonical SMILES |
CC(=O)OCC1=C(N2C(C(C2=O)N)SC1)C(=O)O | |
Source | PubChem | |
URL | https://pubchem.ncbi.nlm.nih.gov | |
Description | Data deposited in or computed by PubChem | |
Isomeric SMILES |
CC(=O)OCC1=C(N2[C@@H]([C@@H](C2=O)N)SC1)C(=O)O | |
Source | PubChem | |
URL | https://pubchem.ncbi.nlm.nih.gov | |
Description | Data deposited in or computed by PubChem | |
Molecular Formula |
C10H12N2O5S | |
Source | PubChem | |
URL | https://pubchem.ncbi.nlm.nih.gov | |
Description | Data deposited in or computed by PubChem | |
DSSTOX Substance ID |
DTXSID9045342 | |
Record name | 7-Aminocephalosporanic acid | |
Source | EPA DSSTox | |
URL | https://comptox.epa.gov/dashboard/DTXSID9045342 | |
Description | DSSTox provides a high quality public chemistry resource for supporting improved predictive toxicology. | |
Molecular Weight |
272.28 g/mol | |
Source | PubChem | |
URL | https://pubchem.ncbi.nlm.nih.gov | |
Description | Data deposited in or computed by PubChem | |
CAS No. |
957-68-6 | |
Record name | 7-Aminocephalosporanic acid | |
Source | CAS Common Chemistry | |
URL | https://commonchemistry.cas.org/detail?cas_rn=957-68-6 | |
Description | CAS Common Chemistry is an open community resource for accessing chemical information. Nearly 500,000 chemical substances from CAS REGISTRY cover areas of community interest, including common and frequently regulated chemicals, and those relevant to high school and undergraduate chemistry classes. This chemical information, curated by our expert scientists, is provided in alignment with our mission as a division of the American Chemical Society. | |
Explanation | The data from CAS Common Chemistry is provided under a CC-BY-NC 4.0 license, unless otherwise stated. | |
Record name | 7-Aminocephalosporanic acid | |
Source | ChemIDplus | |
URL | https://pubchem.ncbi.nlm.nih.gov/substance/?source=chemidplus&sourceid=0000957686 | |
Description | ChemIDplus is a free, web search system that provides access to the structure and nomenclature authority files used for the identification of chemical substances cited in National Library of Medicine (NLM) databases, including the TOXNET system. | |
Record name | 7-Aminocephalosporanic acid | |
Source | EPA DSSTox | |
URL | https://comptox.epa.gov/dashboard/DTXSID9045342 | |
Description | DSSTox provides a high quality public chemistry resource for supporting improved predictive toxicology. | |
Record name | 3-acetoxymethylen-7-amino-8-oxo-5-thia-1-azabicyclo[4.2.0]oct-2-ene-2-carboxylic acid | |
Source | European Chemicals Agency (ECHA) | |
URL | https://echa.europa.eu/substance-information/-/substanceinfo/100.012.259 | |
Description | The European Chemicals Agency (ECHA) is an agency of the European Union which is the driving force among regulatory authorities in implementing the EU's groundbreaking chemicals legislation for the benefit of human health and the environment as well as for innovation and competitiveness. | |
Explanation | Use of the information, documents and data from the ECHA website is subject to the terms and conditions of this Legal Notice, and subject to other binding limitations provided for under applicable law, the information, documents and data made available on the ECHA website may be reproduced, distributed and/or used, totally or in part, for non-commercial purposes provided that ECHA is acknowledged as the source: "Source: European Chemicals Agency, http://echa.europa.eu/". Such acknowledgement must be included in each copy of the material. ECHA permits and encourages organisations and individuals to create links to the ECHA website under the following cumulative conditions: Links can only be made to webpages that provide a link to the Legal Notice page. | |
Record name | 7-AMINOCEPHALOSPORANIC ACID | |
Source | FDA Global Substance Registration System (GSRS) | |
URL | https://gsrs.ncats.nih.gov/ginas/app/beta/substances/9XI67897RG | |
Description | The FDA Global Substance Registration System (GSRS) enables the efficient and accurate exchange of information on what substances are in regulated products. Instead of relying on names, which vary across regulatory domains, countries, and regions, the GSRS knowledge base makes it possible for substances to be defined by standardized, scientific descriptions. | |
Explanation | Unless otherwise noted, the contents of the FDA website (www.fda.gov), both text and graphics, are not copyrighted. They are in the public domain and may be republished, reprinted and otherwise used freely by anyone without the need to obtain permission from FDA. Credit to the U.S. Food and Drug Administration as the source is appreciated but not required. | |
Retrosynthesis Analysis
AI-Powered Synthesis Planning: Our tool employs the Template_relevance Pistachio, Template_relevance Bkms_metabolic, Template_relevance Pistachio_ringbreaker, Template_relevance Reaxys, Template_relevance Reaxys_biocatalysis model, leveraging a vast database of chemical reactions to predict feasible synthetic routes.
One-Step Synthesis Focus: Specifically designed for one-step synthesis, it provides concise and direct routes for your target compounds, streamlining the synthesis process.
Accurate Predictions: Utilizing the extensive PISTACHIO, BKMS_METABOLIC, PISTACHIO_RINGBREAKER, REAXYS, REAXYS_BIOCATALYSIS database, our tool offers high-accuracy predictions, reflecting the latest in chemical research and data.
Strategy Settings
Precursor scoring | Relevance Heuristic |
---|---|
Min. plausibility | 0.01 |
Model | Template_relevance |
Template Set | Pistachio/Bkms_metabolic/Pistachio_ringbreaker/Reaxys/Reaxys_biocatalysis |
Top-N result to add to graph | 6 |
Feasible Synthetic Routes
Q1: What are the advantages of using enzymes in 7-ACA production?
A1: Enzymatic processes offer several advantages over chemical methods, including milder reaction conditions, higher yields, and reduced environmental impact. [, ] For instance, using immobilized DAAO and GLA in a two-step process led to a 72% overall yield of 7-ACA from deacetyl cephalosporin C (DCPC). []
Q2: Are there efforts to further improve the enzymatic production of 7-ACA?
A2: Yes, researchers are actively exploring one-pot enzymatic processes using various enzyme combinations to simplify production and reduce costs. [] These approaches include using cephalosporin C acylase, modified forms of GLA, and combinations of DAAO and GLA, with or without catalase. []
Q3: Can γ-glutamyltranspeptidase (GGT) be used to produce 7-ACA?
A3: While not traditionally used, research has shown that GGT from Bacillus subtilis possesses inherent glutaryl-7-aminocephalosporanic acid acylase activity. [] This activity can be significantly enhanced through protein engineering, potentially offering an alternative route to 7-ACA production. []
Q4: How can the activity of enzymes involved in 7-ACA production be enhanced?
A4: Protein engineering techniques, such as site-directed mutagenesis and directed evolution, are employed to enhance enzyme activity, stability, and substrate specificity. [, ] For example, modifying amino acid residues in GGT that interact with γ-glutamyl compounds or are located in the active center's peripheral region can increase its GL-7-ACA acylase activity. []
Q5: What is the role of immobilization in the enzymatic production of 7-ACA?
A5: Enzyme immobilization offers benefits like enhanced stability, reusability, and ease of separation from the reaction mixture. [, , ] Immobilized DAAO and GLA have demonstrated extended operational stability in the production of 7-ACA. [, ]
Q6: What is the molecular formula and weight of 7-ACA?
A6: The molecular formula of 7-ACA is C8H10N2O3S, and its molecular weight is 214.24 g/mol.
Q7: Is there a spectroscopic method to quantify 7-ACA?
A7: Yes, a colorimetric method has been developed for the determination of 7-ACA and related compounds. [] This method offers a reproducible way to quantify 7-ACA.
Q8: What is the agglomeration phenomenon of 7-ACA crystals, and how does it relate to the crystallization process?
A8: 7-ACA crystals produced using the acid method tend to agglomerate at pH values higher than 1.5, while those produced using the basic method do not. [] This difference is attributed to variations in surface characteristics, such as fractal dimension and wettability, resulting from the different crystallization processes. []
Q9: How does the pH of the solution affect the extraction of 7-ACA?
A9: The extraction of 7-ACA is influenced by the pH of the solution and the type of carrier used. [] Ion-pair extraction with secondary and tertiary amines shows a decrease in distribution coefficient with increasing pH, while ion exchange extraction with quaternary ammonium salts displays the opposite trend. []
Q10: How do cephalosporins, derived from 7-ACA, exert their antibacterial activity?
A10: Cephalosporins, like penicillins, function by inhibiting bacterial cell wall synthesis. [] They interfere with the transpeptidation reaction, preventing the cross-linking of peptidoglycans, essential components of the bacterial cell wall.
Q11: What are the advantages of semisynthetic cephalosporins over naturally occurring ones?
A11: Semisynthetic cephalosporins, derived from 7-ACA, often possess improved pharmacological properties compared to their natural counterparts. [, ] These enhancements may include a broader spectrum of activity, increased potency, improved pharmacokinetic properties, and enhanced stability against bacterial enzymes like beta-lactamases.
Q12: What are activated esters of 7-ACA, and why are they important?
A12: Activated esters of 7-ACA are derivatives designed to react readily with other molecules, enabling the synthesis of a wide array of cephalosporin antibiotics. [] These activated esters provide alternative and potentially more efficient routes to producing existing and novel cephalosporins. []
Q13: What are the challenges associated with the use of 7-ACA derivatives as antibiotics?
A13: The primary challenge is the emergence and spread of bacterial resistance to cephalosporin antibiotics. [, , ] This resistance can arise through various mechanisms, such as the production of β-lactamases, which inactivate cephalosporins by hydrolyzing their β-lactam ring. []
Q14: Is there any research on mitigating the resistance to 7-ACA derived antibiotics?
A14: Yes, research focuses on developing new cephalosporin analogs with improved stability against β-lactamases and exploring combination therapies with β-lactamase inhibitors. [, ]
Q15: What is the future direction of research on 7-ACA?
A15: Future research will likely focus on developing more efficient and sustainable production methods for 7-ACA, engineering enzymes with enhanced catalytic properties, and designing novel cephalosporin analogs with improved activity and reduced susceptibility to resistance mechanisms. [, , ]
試験管内研究製品の免責事項と情報
BenchChemで提示されるすべての記事および製品情報は、情報提供を目的としています。BenchChemで購入可能な製品は、生体外研究のために特別に設計されています。生体外研究は、ラテン語の "in glass" に由来し、生物体の外で行われる実験を指します。これらの製品は医薬品または薬として分類されておらず、FDAから任何の医療状態、病気、または疾患の予防、治療、または治癒のために承認されていません。これらの製品を人間または動物に体内に導入する形態は、法律により厳格に禁止されています。これらのガイドラインに従うことは、研究と実験において法的および倫理的な基準の遵守を確実にするために重要です。