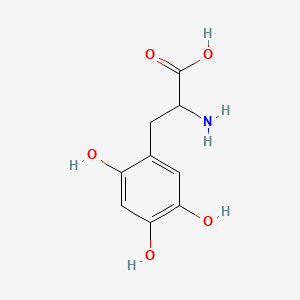
2,4,5-Trihydroxyphenylalanine
説明
2,4,5-Trihydroxyphenylalanine, also known as 6-Hydroxydopa, is a non-proteinogenic alpha-amino acid . It is functionally related to dopa and is a precursor of the neurotoxin 6-hydroxydopamine . It is used to study Parkinson’s disease .
Synthesis Analysis
2,4,5-Trihydroxyphenylalanine can be synthesized from Benzaldehyde, 2,4,5-tris (phenylmethoxy)- . There are also modified syntheses of 2,4,5-trihydroxyphenylalanine .Molecular Structure Analysis
The molecular formula of 2,4,5-Trihydroxyphenylalanine quinone is C15H15NO7 . Its average mass is 321.282 Da and its monoisotopic mass is 321.084839 Da .Chemical Reactions Analysis
2,4,5-Trihydroxyphenylalanine plays a role in melanin biosynthesis . Both 3,4-dihydroxyphenylalanine and 2,4,5-trihydroxyphenylalanine were oxidized with periodate and mushroom of 2-carboxy-2,3-dihydroindole-5,6-quinone reduction .Physical And Chemical Properties Analysis
The molecular formula of 2,4,5-Trihydroxyphenylalanine quinone is C15H15NO7 . Its average mass is 321.282 Da and its monoisotopic mass is 321.084839 Da .科学的研究の応用
Allosteric Inhibitor of RAD52
6-Hydroxy-DL-DOPA acts as an allosteric inhibitor of RAD52 . It inhibits RAD52 binding to single-strand DNA binding domains . RAD52 plays a crucial role in DNA repair, and its inhibition can have significant implications in the study of DNA repair mechanisms and related diseases.
Inhibition of BRCA-deficient Cancer Cells
This compound selectively inhibits the proliferation of BRCA-deficient cancer cells in vitro . BRCA genes are involved in repairing damaged DNA and play an important role in maintaining the genetic stability of cells. When these genes are mutated, they may lead to certain types of cancer, including breast and ovarian cancers.
Inhibitor of APE1
6-Hydroxy-DL-DOPA also inhibits APE1 , a key enzyme involved in the DNA base excision repair pathway. This pathway is responsible for repairing small, non-helix-distorting base lesions from the genome. The inhibition of APE1 can be significant in the study of DNA repair mechanisms.
Research Tool in Cancer Studies
Given its inhibitory effects on RAD52 and APE1, as well as its selective inhibition of BRCA-deficient cancer cells, 6-Hydroxy-DL-DOPA can be used as a research tool in cancer studies .
DNA, RNA, and Protein Synthesis Inhibitor
6-Hydroxy-DL-DOPA is listed among DNA, RNA, and Protein Synthesis Inhibitors . This suggests that it may have a role in inhibiting the synthesis of these crucial biological molecules, although the specific mechanisms and implications need further research.
作用機序
Target of Action
6-Hydroxy-DL-DOPA, also known as 6-Hydroxydopa or 2,4,5-Trihydroxyphenylalanine, primarily targets two proteins: RAD52 and APE1 .
- RAD52 : This protein plays a crucial role in DNA repair, particularly in the homologous recombination pathway .
- APE1 : Also known as apurinic/apyrimidinic endonuclease, APE1 is a nuclease that is essential for the DNA base excision repair pathway .
Mode of Action
6-Hydroxy-DL-DOPA acts as an allosteric inhibitor of RAD52, inhibiting RAD52’s binding to single-strand DNA binding domains . It also inhibits the repair-function activity of APE1 .
Biochemical Pathways
The primary biochemical pathways affected by 6-Hydroxy-DL-DOPA are the DNA repair pathways . By inhibiting RAD52 and APE1, 6-Hydroxy-DL-DOPA disrupts the homologous recombination pathway and the DNA base excision repair pathway, respectively .
Pharmacokinetics
It is soluble in 1m hcl, suggesting that it may be absorbed in the acidic environment of the stomach .
Result of Action
The inhibition of RAD52 and APE1 by 6-Hydroxy-DL-DOPA leads to a disruption in DNA repair, which can result in DNA damage and genomic instability . This compound selectively inhibits the proliferation of BRCA-deficient cancer cells in vitro .
Action Environment
It is known that the compound should be stored at -20°c and that solutions should be freshly prepared and protected from exposure to light .
将来の方向性
The structure of a new biological redox cofactor – topaquinone (TPQ), the quinone of 2,4,5-trihydroxyphenylalanine – was elucidated in 1990 . TPQ is the cofactor in most copper-containing amine oxidases . It is produced by post-translational modification of a strictly conserved active-site tyrosine residue . This suggests potential future directions in the study of this compound and its role in biological processes.
特性
IUPAC Name |
2-amino-3-(2,4,5-trihydroxyphenyl)propanoic acid | |
---|---|---|
Source | PubChem | |
URL | https://pubchem.ncbi.nlm.nih.gov | |
Description | Data deposited in or computed by PubChem | |
InChI |
InChI=1S/C9H11NO5/c10-5(9(14)15)1-4-2-7(12)8(13)3-6(4)11/h2-3,5,11-13H,1,10H2,(H,14,15) | |
Source | PubChem | |
URL | https://pubchem.ncbi.nlm.nih.gov | |
Description | Data deposited in or computed by PubChem | |
InChI Key |
YLKRUSPZOTYMAT-UHFFFAOYSA-N | |
Source | PubChem | |
URL | https://pubchem.ncbi.nlm.nih.gov | |
Description | Data deposited in or computed by PubChem | |
Canonical SMILES |
C1=C(C(=CC(=C1O)O)O)CC(C(=O)O)N | |
Source | PubChem | |
URL | https://pubchem.ncbi.nlm.nih.gov | |
Description | Data deposited in or computed by PubChem | |
Molecular Formula |
C9H11NO5 | |
Source | PubChem | |
URL | https://pubchem.ncbi.nlm.nih.gov | |
Description | Data deposited in or computed by PubChem | |
DSSTOX Substance ID |
DTXSID00943928 | |
Record name | 2,5-Dihydroxytyrosine | |
Source | EPA DSSTox | |
URL | https://comptox.epa.gov/dashboard/DTXSID00943928 | |
Description | DSSTox provides a high quality public chemistry resource for supporting improved predictive toxicology. | |
Molecular Weight |
213.19 g/mol | |
Source | PubChem | |
URL | https://pubchem.ncbi.nlm.nih.gov | |
Description | Data deposited in or computed by PubChem | |
Product Name |
2,4,5-Trihydroxyphenylalanine | |
CAS RN |
21373-30-8 | |
Record name | 3-(2,4,5-Trihydroxyphenyl)-DL-alanine | |
Source | CAS Common Chemistry | |
URL | https://commonchemistry.cas.org/detail?cas_rn=21373-30-8 | |
Description | CAS Common Chemistry is an open community resource for accessing chemical information. Nearly 500,000 chemical substances from CAS REGISTRY cover areas of community interest, including common and frequently regulated chemicals, and those relevant to high school and undergraduate chemistry classes. This chemical information, curated by our expert scientists, is provided in alignment with our mission as a division of the American Chemical Society. | |
Explanation | The data from CAS Common Chemistry is provided under a CC-BY-NC 4.0 license, unless otherwise stated. | |
Record name | 6-Hydroxydopa | |
Source | ChemIDplus | |
URL | https://pubchem.ncbi.nlm.nih.gov/substance/?source=chemidplus&sourceid=0021373308 | |
Description | ChemIDplus is a free, web search system that provides access to the structure and nomenclature authority files used for the identification of chemical substances cited in National Library of Medicine (NLM) databases, including the TOXNET system. | |
Record name | 2,5-Dihydroxytyrosine | |
Source | EPA DSSTox | |
URL | https://comptox.epa.gov/dashboard/DTXSID00943928 | |
Description | DSSTox provides a high quality public chemistry resource for supporting improved predictive toxicology. | |
Record name | 6-HYDROXYDOPA, DL- | |
Source | FDA Global Substance Registration System (GSRS) | |
URL | https://gsrs.ncats.nih.gov/ginas/app/beta/substances/9HBL9XYQ8O | |
Description | The FDA Global Substance Registration System (GSRS) enables the efficient and accurate exchange of information on what substances are in regulated products. Instead of relying on names, which vary across regulatory domains, countries, and regions, the GSRS knowledge base makes it possible for substances to be defined by standardized, scientific descriptions. | |
Explanation | Unless otherwise noted, the contents of the FDA website (www.fda.gov), both text and graphics, are not copyrighted. They are in the public domain and may be republished, reprinted and otherwise used freely by anyone without the need to obtain permission from FDA. Credit to the U.S. Food and Drug Administration as the source is appreciated but not required. | |
Retrosynthesis Analysis
AI-Powered Synthesis Planning: Our tool employs the Template_relevance Pistachio, Template_relevance Bkms_metabolic, Template_relevance Pistachio_ringbreaker, Template_relevance Reaxys, Template_relevance Reaxys_biocatalysis model, leveraging a vast database of chemical reactions to predict feasible synthetic routes.
One-Step Synthesis Focus: Specifically designed for one-step synthesis, it provides concise and direct routes for your target compounds, streamlining the synthesis process.
Accurate Predictions: Utilizing the extensive PISTACHIO, BKMS_METABOLIC, PISTACHIO_RINGBREAKER, REAXYS, REAXYS_BIOCATALYSIS database, our tool offers high-accuracy predictions, reflecting the latest in chemical research and data.
Strategy Settings
Precursor scoring | Relevance Heuristic |
---|---|
Min. plausibility | 0.01 |
Model | Template_relevance |
Template Set | Pistachio/Bkms_metabolic/Pistachio_ringbreaker/Reaxys/Reaxys_biocatalysis |
Top-N result to add to graph | 6 |
Feasible Synthetic Routes
Q & A
Q1: How does 6-hydroxydopa (TOPA) exert its neurotoxic effects?
A1: TOPA's neurotoxicity is primarily attributed to two mechanisms:
- Excitotoxicity: TOPA, particularly its oxidized form, topa quinone, acts as a potent non-N-methyl-D-aspartate (non-NMDA) glutamatergic agonist. [] This leads to excessive neuronal excitation and subsequent cell death. [, ]
- Oxidative Stress: TOPA readily undergoes autoxidation, generating reactive oxygen species (ROS) such as hydrogen peroxide (H2O2), superoxide radical (O2•-), and hydroxyl radical (•OH). [, ] These ROS cause oxidative damage to cellular components like lipids, proteins, and DNA, ultimately contributing to neuronal death. [, ]
Q2: Does 6-hydroxydopamine (6-OHDA) play a role in TOPA-induced neurotoxicity?
A2: While TOPA itself is neurotoxic, its in vivo conversion to 6-OHDA might contribute to its overall toxicity. 6-OHDA is a well-known neurotoxin that causes degeneration of noradrenergic nerve terminals. [] Some studies suggest that TOPA might be converted to 6-OHDA in vivo, further exacerbating its neurotoxic effects. []
Q3: How does TOPA affect norepinephrine (NE) levels in the brain?
A3: TOPA administration leads to a complex pattern of NE alterations in different brain regions:
- Depletion: In regions supplied by the dorsal noradrenergic bundle, like the neocortex and hippocampus, TOPA causes significant and long-lasting NE depletion. [] This depletion is likely due to the degeneration of noradrenergic neurons and impaired NE uptake. []
- Elevation: Conversely, TOPA can elevate NE levels in other areas like the cerebellum. [, ] This increase is attributed to enhanced intraneuronal NE storage rather than increased nerve endings. []
Q4: What is the molecular formula and weight of 6-hydroxydopa?
A4: The molecular formula of 6-hydroxydopa is C9H11NO5, and its molecular weight is 213.19 g/mol.
Q5: What are the key spectroscopic characteristics of 6-hydroxydopa and its derivatives?
A5:
- 6-Hydroxydopa: Exhibits a UV absorbance maximum (λmax) at 291 nm. []
- Topa Quinone: Displays a UV absorbance maximum (λmax) at 460 nm in the presence of amines. []
- 6-Hydroxydopa Radical: Characterized by a sharp peak at 440 nm, shifting to 460 nm upon amine addition. []
- Phenylhydrazine and p-Nitrophenylhydrazine Derivatives: Exhibit distinct resonance Raman spectra that are consistent with the presence of topa quinone. []
Q6: What is the biological role of 6-hydroxydopa?
A6: TOPA, in its oxidized quinone form (TPQ), serves as an essential cofactor in copper amine oxidases (CAOs). [, , , ] CAOs are enzymes that catalyze the oxidative deamination of primary amines. []
Q7: How does topa quinone function as a cofactor in CAOs?
A7: Topa quinone acts as a redox-active cofactor, cycling between its oxidized (quinone) and reduced (benzenetriol) forms during the catalytic cycle of CAOs. It participates in the transfer of electrons from the amine substrate to molecular oxygen, ultimately leading to the formation of an aldehyde, ammonia, and hydrogen peroxide. [, , , ]
Q8: Can copper amine oxidases function without the topa quinone cofactor?
A8: No, the topa quinone cofactor is essential for the catalytic activity of copper amine oxidases. Studies with copper-free apoenzymes demonstrate that they cannot oxidize substrates efficiently. [, ]
Q9: How is topa quinone generated in CAOs?
A9: Topa quinone is produced through post-translational modification of a specific tyrosine residue within the CAO active site. This process requires both molecular oxygen and the enzyme-bound copper ion. [, , ]
Q10: What are the main toxic effects of 6-hydroxydopa?
A10: TOPA is a strong neurotoxin, primarily targeting catecholaminergic neurons. [, , ] Its toxicity is linked to both its ability to induce oxidative stress [, ] and its action as an excitotoxic agent, particularly in its oxidized topa quinone form. [, ]
Q11: Are there any protective strategies against TOPA-induced neurotoxicity?
A11: Research suggests that certain compounds may offer protection against TOPA-induced damage:
- Antioxidants: Compounds like norepinephrine can neutralize the cytotoxic effects of TOPA and its related compounds by scavenging free radicals generated during autoxidation. []
- NMDA Receptor Antagonists: Antagonists of non-NMDA glutamate receptors, such as 6-cyano-7-nitroquinoxaline-2,3-dione (CNQX), can block TOPA's excitotoxic effects and protect neurons from cell death. [, , ]
- Ganglioside GM1: This neuroprotective agent has demonstrated efficacy in safeguarding neurons against TOPA-induced degeneration. []
試験管内研究製品の免責事項と情報
BenchChemで提示されるすべての記事および製品情報は、情報提供を目的としています。BenchChemで購入可能な製品は、生体外研究のために特別に設計されています。生体外研究は、ラテン語の "in glass" に由来し、生物体の外で行われる実験を指します。これらの製品は医薬品または薬として分類されておらず、FDAから任何の医療状態、病気、または疾患の予防、治療、または治癒のために承認されていません。これらの製品を人間または動物に体内に導入する形態は、法律により厳格に禁止されています。これらのガイドラインに従うことは、研究と実験において法的および倫理的な基準の遵守を確実にするために重要です。